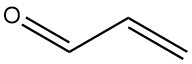
Acrolein, also known as propenal or acrylaldehyde, is the simplest unsaturated aldehyde with the formula C3H4O. It is a volatile, colorless liquid with a strong odor that is both toxic and tear-inducing.
The discovery of acrolein, which derives its name from the Latin words “acer” meaning acrid and “oleum” meaning oil, can be attributed to Redtenbacher in 1843. It was found in overheated fat.
In 1942, Degussa established a method for commercially producing acrolein through the heterogeneously catalyzed gas-phase condensation of acetaldehyde and formaldehyde. Presently, acrolein is predominantly manufactured on a large scale by the heterogeneously catalyzed gas-phase oxidation of propene.
Table of Contents
1. Physical Properties of Acrolein
Acrolein has limited solubility in water but is soluble in various organic solvents, including alcohols, ethers, and aliphatic or aromatic hydrocarbons. The essential physical properties of acrolein are presented below:
Property | Value |
---|---|
Molar Mass | 56.06 g/mol |
Boiling Point (bp) | 52-53°C |
Melting Point (mp) | -87°C |
Relative Density | 0.838 g/cm³ |
Refractive Index | 1.418 |
Viscosity | 0.40 cP at 25°C |
Solubility in Water | 260 g/kg |
Vapor Pressure | 29 kPa at 20°C |
Autoignition Temperature in Air | 234 °C |
Flash Point | -26 °C |
2. Chemical Reactions of Acrolein
Acrolein exhibits high reactivity due to its conjugated vinyl and aldehyde groups, making it undergo reactions characteristic of both unsaturated compounds and aldehydes.
The conjugation between the carbon-carbon double bond and the carbonyl group enhances the reactivity of both groups, allowing them to react together or separately.
It is worth noting that acrolein can undergo spontaneous, highly exothermic polymerization.
2.1. Diels-Alder Reaction
Acrolein acts as both a diene and a dienophile, enabling the formation of a cyclic dimer called 3,4-dihydro-2H-pyran-2-carboxaldehyde. This dimer is produced through the uncatalyzed thermal reaction of acrolein at approximately 190 °C (1 hour for 75% acrolein conversion), along with the formation of polymeric side products.
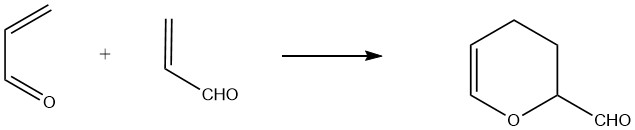
To achieve high yields, stabilization with hydroquinone or complexing compounds like polyvalent organic acids is necessary.
The resulting “thermal dimer” is a clear liquid with an unpleasant odor, boiling at 151.3 °C at 101.3 kPa and having a relative density of 1.0775. Acid hydrolysis of the dimer yields 2-hydroxyadipaldehyde, which can be further hydrogenated to form 1,2,6-hexanetriol.
Acrolein acts as a 1,3-diene in reactions with dienophiles where the electron density of the carbon-carbon double bond is increased by electron-releasing substituents. For instance, acrolein readily reacts with vinyl ethers and vinylamines to form dihydropyrans.
A significant commercial example is the reaction between methyl vinyl ether and acrolein, leading to the formation of 3,4-dihydro-2-methoxy-2H-pyran. Reported yields for this reaction at temperatures of 160-190 °C range from 80% to 90%.
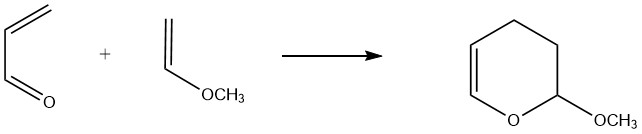
2.2. Addition to the Carbon-Carbon Double Bond
The β-carbon atom of acrolein, polarized by the carbonyl group, acts as an electrophile. Consequently, nucleophilic reagents such as alcohols, thiols, water, amines, active methylene compounds, and inorganic and organic acids can add to the carbon-carbon double bond in the presence of acidic or basic catalysts.
Carefully controlled conditions are essential to minimize undesirable side reactions. The addition of water under mild acidic conditions leads to the formation of 3-hydroxypropionaldehyde (HPA) with high selectivity.
Buffer solutions with a pH of 4-5 or weak acidic ion-exchange resins are often used as catalysts. Further hydrogenation of the aqueous solutions results in 1,3-propanediol.
Precious metal catalysts facilitate the direct oxidation of aqueous solutions of HPA at pH 3, producing 3-hydroxypropionic acid. Above pH 7, the malonate anion is formed in high yield.
Acrolein reacts rapidly with hydrogen chloride or hydrogen bromide to yield 3-chloropropionaldehyde or 3-bromopropionaldehyde. However, these products readily polymerize into trimers and tetramers in the presence of acids. To obtain 3-halopropionaldehyde acetals, a simultaneous hydrogen halide addition and acetalization process is preferred, resulting in acetal yields of approximately 90%.
Chlorine and bromine can add to acrolein in dilute aqueous solution, forming 2,3-dihalopropionaldehydes with yields of around 85%. These dihalopropionaldehydes can be dehydrohalogenated to produce 2-haloacroleins. The 2-haloacroleins are known to be potent mutagens and can be further halogenated to yield 2,2,3-trihalopropionaldehydes, which are valuable intermediates in folic acid synthesis.
Hydrogen sulfide, when added to two equivalents of acrolein followed by an aldol reaction, results in the formation of 3-formyl-5,6-dihydrothiopyran.

Methanethiol undergoes base-catalyzed addition to acrolein, leading to the formation of 3-(methylthio)propionaldehyde, which is an important reaction used in the synthesis of the essential amino acid D,L-methionine.
2.3. Reactions of the Aldehyde Group
The selectivity of acid-catalyzed acetalization depends on the nature of the alcohol used and the catalysts employed. Side reactions can produce corresponding 3-alkoxypropionaldehydes and 3-alkoxypropionaldehyde acetals.
Cyclic acetals, particularly those formed with branched diols like 2-methyl-1,3-propanediol, are easier to obtain compared to acyclic acetals of lower alcohols. Various continuous processes are known for the preparation of cyclic acetals, with yields of more than 90% achievable through special processes in combination with extraction.
Acrolein acetals serve as valuable intermediates and have gained interest as safer forms for the transport of acrolein, especially 2-vinyl-1,3-dioxolane. These acetals can be easily cleaved back into acrolein in aqueous acidic solutions, which find utility in the use of acrolein as an aquatic herbicide and as a hydrogen sulfide scavenger in oil-field waters.
Spiro diacetal, diallylidene pentaerythritol, prepared by condensing pentaerythritol with two moles of acrolein, can be polymerized with vinyl or other monomers to form spirane resins.
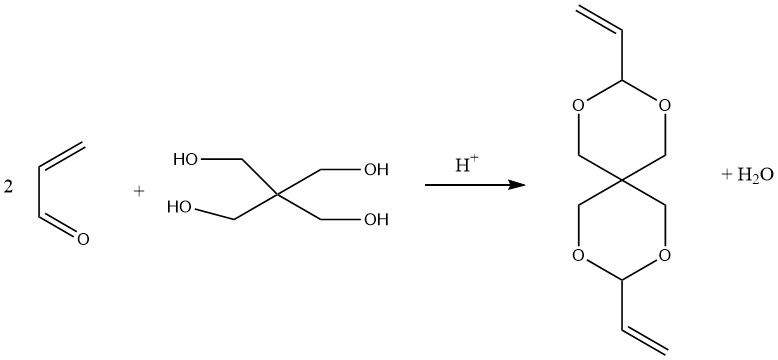
Hydroformylation of acrolein acetals, particularly cyclic acetals, leads to the monoacetals of 1,4-butanedial. Subsequent hydrolysis and hydrogenation of this hydroformylation product result in the production of 1,4-butanediol.
Acrolein diacetate, also known as 2-propene-1,1-diol diacetate, is easily prepared in 90% yield by the acid-catalyzed reaction of acetic anhydride with acrolein.
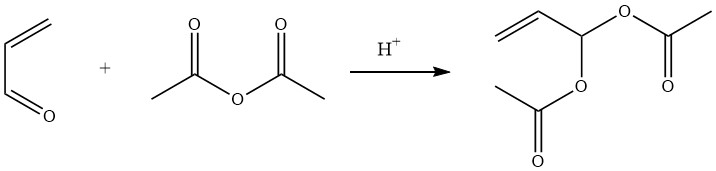
When hydrogen cyanide is added to acrolein in the presence of mild alkaline catalysts, acrolein cyanohydrin is formed with yields exceeding 90%. Acrolein cyanohydrin acetate, obtained through its reaction with acetic anhydride, serves as a valuable intermediate in the synthesis of pharmaceutically and biologically active substances.
2.4. Simultaneous Reaction of the Aldehyde and Vinyl Groups
Although acrolein and methacrolein are recognized as intermediates in the Skraup synthesis of quinolines from aromatic amines and glycerol, their commercial utilization as starting materials for this reaction remains limited.
The gas-phase condensation of acrolein and ammonia, catalyzed by multicomponent Al2O3 or SiO2-Al2O3 catalysts, yields 45% 3-methylpyridine and 20-25% pyridine as a by product. This reaction has been generalized as a universal synthesis for substituted pyridines.

Acrolein reacts with isocyanuric acid to form an adduct useful as a cross-linking agent. The bifunctional reactivity of acrolein has been exploited in various syntheses of heterocycles, such as the reactions with phenols to yield substituted chromenes or chromanes, and the reaction with 2-aminophenols to produce 8-quinolinols.
When acrolein is reacted with excess phenol under acidic conditions, a mixture of polyphenols is obtained, with 1,1,3-tris(4-hydroxyphenyl)propane as the primary product.
Additionally, acrolein reacts with urea to yield hexahydro-2-oxo-4-pyrimidinyl urea, which has been proposed as a fertilizer. The condensation of acrolein with hydrazine and substituted hydrazines gives pyrazolines in 80% yield.
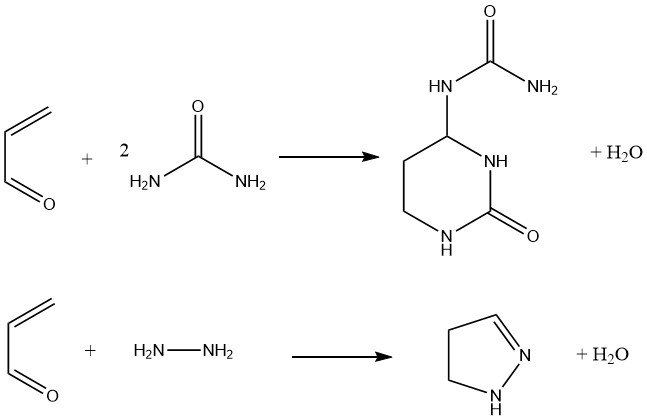
Acrolein can be reacted with formaldehyde under alkaline conditions to produce pentaerythritol. However, the yields are lower compared to the commercial synthesis of pentaerythritol via acetaldehyde and formaldehyde.
Sodium bisulfite reacts with acrolein to form a stable adduct called the disodium salt of 1-hydroxypropane-1,3-disulfonic acid. This reaction finds convenient application for deodorization of spilled acrolein.
2.5. Reduction
Selective reduction of acrolein to allyl alcohol in high yields (90%) can be achieved through hydrogen transfer from secondary alcohols, such as 2-propanol, in a Meerwein-Ponndorf reaction in the liquid phase.
In the gas phase, hydrogen transfer from ethanol or 2-propanol over magnesium oxide catalysts or rare earth oxide catalysts at temperatures ranging from 300 to 500 °C also leads to the production of allyl alcohol.

However, catalytic hydrogenation of acrolein is less selective or only selective at very low conversions.
2.6. Oxidation
By reacting acrolein with aqueous hydrogen peroxide at pH 8-8.5, glycidaldehyde can be obtained in yields of 80-90%:

Hydration of the oxirane group in glycidaldehyde leads to an aqueous solution of D,L-glyceraldehyde.
Acrylic acid is commercially produced through the oxidation of acrolein with molecular oxygen.
2.7. Polymerization
Acrolein readily polymerizes when heated, exposed to light, or in the presence of various initiators. The properties of the resulting acrolein polymer depend on the polymerization conditions, such as the initiator type, solvent, and reaction temperature.
The functional groups of acrolein (vinyl and aldehyde) can polymerize separately or together. Radical-initiated polymerization occurs exclusively at the vinyl group, resulting in polymers with aldehyde groups that readily form tetrahydropyran structures. Ionic polymerization mainly yields polymers with vinyl groups, alongside polymers containing both aldehyde and vinyl groups.
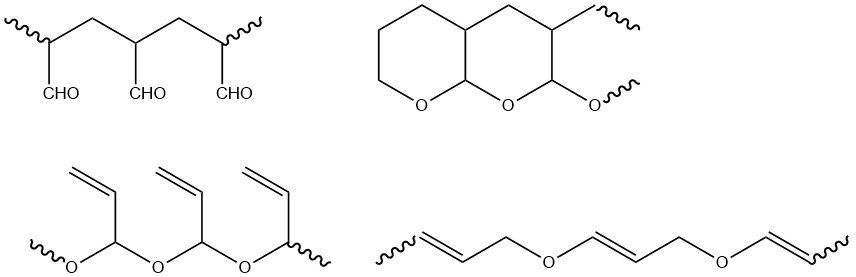
Radical polymers are infusible and insoluble in common solvents, while products of anionic initiation are soluble in many organic solvents. Apart from homopolymers, copolymers with various vinylic and acrylic comonomers have been synthesized.
The carbonyl groups of polyacrolein can be chemically modified under mild conditions. Many polyacrolein derivatives are soluble in organic solvents or even in water. These polymers have attracted interest due to their biocidal activity, as acrolein is slowly released from them.
The photochemical (laser) method has been utilized to synthesize ultrafine particles from gaseous mixtures of acrolein and certain organosilicon compounds. Polyacrolein particles are being explored for their potential applications in nanotechnology.
3. Production of Acrolein
3.1. Acrolein by Propene Oxidation
The first commercial process for acrolein production was developed by Degussa in 1942. It involved the vapor-phase condensation of acetaldehyde and formaldehyde, catalyzed by sodium silicate on silica supports at temperatures of 300-320 °C.
This method was used until 1959 when Shell introduced a new process based on the vapor-phase oxidation of propene over a cuprous oxide catalyst. However, the catalyst performance in this process was poor.
In 1957, Standard Oil of Ohio (Sohio) discovered the bismuth molybdate catalyst system, which showed improved selectivity but still had low propene conversion.
The oxidation of propene to acrolein gained momentum with the discovery of the bismuth molybdate-bismuth phosphomolybdate system (Bi9PMo12O52 on an SiO2 support) by Sohio. Commercial catalysts also include bismuth-molybdenum oxides.
The catalysis of propene oxidation to acrolein involves two reaction cycles: a catalyst reduction cycle (selective product formation) and a catalyst reoxidation cycle (lattice-oxygen regeneration). The active sites of the catalysts are interfaces between Fe2(MoO4)3 and β-CoMoO4, with bismuth molybdate being responsible for the selectivity.
Modern catalysts for acrolein production are multicomponent metal oxide systems.
The optimization of these catalyst systems is an ongoing challenge, with various chemical companies continuously working on improving catalyst compositions and preparation techniques. Additional metal oxides such as Co, Ni, P, Sb, W, or K are added to balance the redox properties of the catalyst and enhance its performance.
In the current production processes, propene is mixed with air and steam and fed to a multi-tubular fixed-bed reactor operated at temperatures of 300-400 °C. The reactor effluent is then quenched, and the gas is scrubbed with water or water/solvent mixtures to remove byproducts.
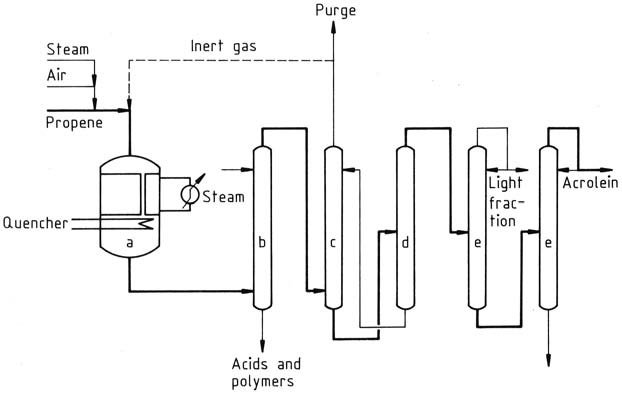
a) Oxidation reactor; b) Scrubber; c) Absorber; d) Desorber; e) Fractionators
The acrolein is obtained by absorbing the gas in cold water in an absorber. The aqueous solution of acrolein is further processed in a desorption column to obtain crude acrolein, which is then distilled to purify the product.
New developments in acrolein production focus on improving catalyst selectivity and yield while reducing energy consumption and CO2 emissions.
Alternative routes to acrolein from propane are being explored, and the combination of propane dehydrogenation and selective oxidation of propene to acrolein is being investigated.
The Methanol to Propene process, using methanol as a feedstock for propene production, is also gaining importance in the chemical industry.
4. Uses of Acrolein
Acrolein has a wide range of uses as a chemical intermediate in various industries. Some of the major uses of acrolein include:
1. Biocide: Acrolein is used as a highly effective broad-spectrum biocide at low concentrations, around 10 ppm. It is used to control the growth of aquatic weeds, algae, mollusks, and as a disinfectant in water systems. It is also used in oil-field waters to eliminate malodorous hydrogen sulfide.
2. Methionine Production: Acrolein is primarily used in the production of methionine, which is an essential amino acid and an animal feed supplement. Acrolein is also used to produce methionine hydroxy analogue, which is a feed supplement with slightly lower bioefficacy than methionine.
3. Acrylic Acid: Acrolein is a precursor for the production of acrylic acid, which is an important chemical used to make acrylates. Acrylic acid is widely used in the manufacture of coatings, adhesives, textiles, and various polymers.
4. 1,3-Propanediol: Acrolein can be used to produce 1,3-propanediol through a hydrolysis and hydrogenation process. 1,3-Propanediol finds applications in the production of composite materials, adhesives, solvents, and anti-freeze.
5. Glutaraldehyde: Acrolein is used in the production of glutaraldehyde, which is a versatile dialdehyde. Glutaraldehyde is used in leather tanning, water treatment, oil-field applications, and as a disinfectant and sterilizer for medical equipment.
6. Pyridines: Acrolein can be used to synthesize various pyridine derivatives, including 3-methylpyridine and other substituted pyridines, which have applications in the pharmaceutical, pesticide, and fragrance industries.
7. Tetrahydrobenzaldehyde: Acrolein can be used in the production of tetrahydrobenzaldehyde, which is used in the synthesis of pharmaceuticals, fungicides, and fragrances.
8. Flavors and Fragrances: Acrolein and its derivatives are used in the production of a wide range of flavors and fragrances, including compounds like lyral, myrac aldehyde, and 5-norbornene-2-carbaldehyde.
9. Herbicides: Acrolein cyanohydrin acetate is used as an intermediate in the production of phosphinotrycin, a nonselective herbicide used in crop protection.
10. Other Applications: Acrolein has been used in the past for the production of allyl alcohol and glycerol, but these processes have been discontinued. It can also be used to produce D,L-glyceraldehyde, which has applications in leather tanning and various syntheses. Acrolein polymers, such as poly(aldehyde carboxylic acid), have industrial applications as sequestering agents, textile treatments, paper reinforcement, and biocides.
These are just some of the major uses of acrolein, and its versatility as a chemical intermediate allows for potential applications in various industries.
Reference
- Acrolein and Methacrolein; Ullmann’s Encyclopedia of Industrial Chemistry. – https://onlinelibrary.wiley.com/doi/10.1002/14356007.a01_149.pub2
FAQ: Acrolein
Acrolein is a volatile organic compound (VOC) that is a colorless liquid with a pungent odor. It is an unsaturated aldehyde with the chemical formula C3H4O.
Acrolein has various industrial applications. It is primarily used as a chemical intermediate for the production of methionine, acrylic acid, 1,3-propanediol, glutaraldehyde, pyridines, flavors, and fragrances. It is also used as a biocide in water treatment, oil-field applications, and as a disinfectant for medical equipment.
Acrolein can be formed through the vapor-phase condensation of acetaldehyde and formaldehyde, catalyzed by sodium silicate on silica supports. It can also be produced by the vapor-phase oxidation of propene over a cuprous oxide catalyst.
Yes, acrolein is toxic. It is considered a highly irritating and corrosive compound. Inhalation or exposure to acrolein can cause respiratory irritation, eye irritation, skin burns, and other health effects. It is important to handle acrolein with proper safety precautions and in well-ventilated areas.
When working with acrolein, it is essential to wear appropriate personal protective equipment (PPE). This typically includes chemical-resistant gloves, goggles or a face shield, a lab coat or chemical-resistant clothing, and closed-toe shoes. A respirator may be required depending on the concentration and duration of exposure.
Acrolein can be found in various sources, both natural and anthropogenic. It can be formed during the combustion of organic matter, such as tobacco smoke, vehicle exhaust, and industrial emissions. It is also produced by certain plants as a defense mechanism. Additionally, acrolein can be present in some foods, particularly during the heating or frying of fats and oils.