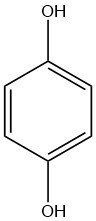
Hydroquinone, also known as 1,4-benzenediol, is a colorless crystalline solid when pure, with the chemical formula C6H4(OH)2. It is an aromatic diol (a para-isomer of catechol).
The discovery of hydroquinone dates back to 1844, when WOEHLER first reported its existence. It is a colorless crystalline solid that is synthesized by adding hydrogen to 1,4-benzoquinone.
Hydroquinone is also found in nature as hydroquinone β-D-glucopyranoside (arbutin) in various plants, such as bearberry, cranberry, cowberry, and certain types of pear, often alongside its methyl ether, methylarbutin.
Under hot, dilute aqueous acid conditions, arbutin is easily hydrolyzed to hydroquinone and glucose. Similarly, the hydrolysis of methylarbutin results in the formation of hydroquinone monomethyl ether and glucose.
Table of Contents
1. Production of hydroquinone
Three processes are used for the industrial production of hydroquinone: hydroperoxidation of p-diisopropylbenzene, hydroxylation of phenol, and oxidation of aniline.
1.1. Hydroperoxidation of p-Diisopropylbenzene
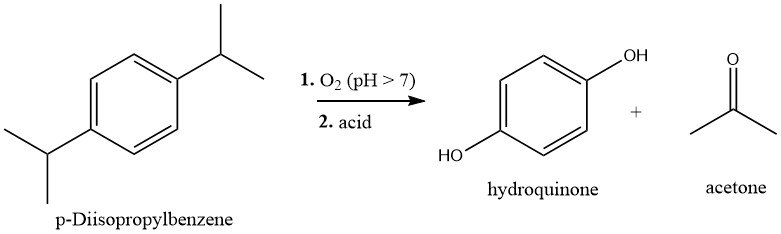
p-Diisopropylbenzene (p-DIPB) is synthesized by the Friedel-Crafts alkylation of benzene with propene. The resulting p-DIPB is purified and then oxidized with air under slightly alkaline conditions at 80-90°C to form dihydroperoxide (DHP).
The DHP can be extracted from the reaction mixture or obtained through crystallization, and subsequently cleaved to produce hydroquinone and acetone by an acid-catalyzed Hock rearrangement. This process involves treating the DHP solution with sulfuric acid catalyst (0.2-1.0%) at 60-80°C. The hydroquinone is then isolated and crystallized.
The overall yield of hydroquinone, based on p-DIPB, is approximately 80%.
1.2. Hydroxylation of Phenol
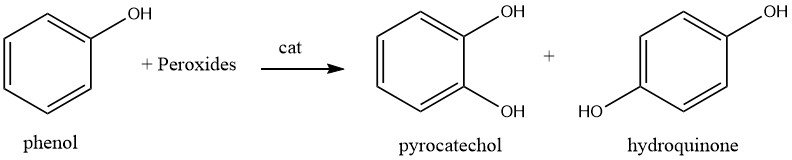
The hydroxylation of phenol using hydrogen peroxide at a temperature of around 80 °C and a catalyst such as a strong mineral acid, iron(II), or cobalt(II) salt, results in a mixture of hydroquinone and catechol. The ratio of catechol to hydroquinone can be varied from 3:1 to 0.1:1, with a typical ratio of 1.5:1 where catechol is the major product.
The mechanism of the reaction is ionic in nature, whereby hydrogen peroxide is polarized by the strong acid catalyst and phenol is subsequently hydroxylated. The resulting isomers are separated by a series of extractions and solvent-stripping operations.
The ratio of hydroquinone and catechol products can be affected by the presence of superacids or shape-selective zeolites. For example, the use of a vanadium-modified Nafion perfluorosulfonate polymer in the oxidation of phenol resulted in a hydroquinone-catechol ratio of 12.5:1.
The use of a shape-selective zeolite in the hydroxylation of phenol led to a hydroquinone selectivity of 99%.
1.3. Oxidation of Aniline
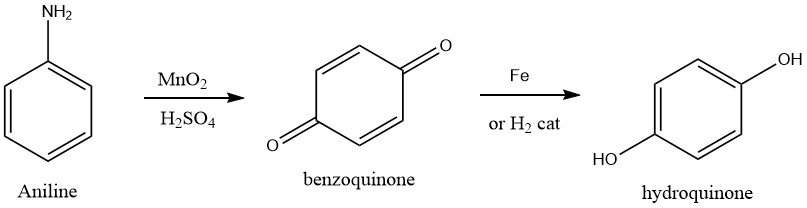
The production of hydroquinone has been historically accomplished by aniline oxidation, which is considered the oldest known process. To achieve this, aniline is oxidized with a 15-20% excess of manganese dioxide in an aqueous sulfuric acid solution maintained at 0-5 °C.
This reaction yields p-benzoquinone, which is separated from the reaction mixture via steam stripping and collected. The reaction produces a byproduct, manganese sulfate, which can be obtained from the depleted reaction mixture and sold for agricultural purposes.
Hydroquinone is then obtained from the p-benzoquinone intermediate through reduction with iron at 55-65 °C or by catalytic hydrogenation. Typically, the technical-grade product is crystallized, isolated from the aqueous stream via centrifugation, and dried using a vacuum dryer. The overall yield of hydroquinone from aniline is approximately 85%.
Aniline oxidation is a batchwise process, and as such, it is relatively labor-intensive. Processing conditions are also highly abrasive because ground manganese ore and finely divided iron are used, wich demande a constant maintenance.
The disposal of inorganic co-products, which constitute about 85% of the total weight of the products, poses a major environmental problem.
1.4. Other Processes
Baeyer-Villiger oxidation of a 4-hydroxy-substituted aromatic ketone gives carboxylate esters of aromatic diols with high yield and efficiency of 97%.
Microbiological oxidation of benzene or phenol is highly selective and produces hydroquinone with remarkable selectivity.
Air oxidation of phenol in the presence of copper catalyst provides p-benzoquinone in selectivity greater than 90%. Oxidation of benzene to hydroquinone can be achieved using copper(I) chloride or titanium.
Ozone-induced oxidation of benzene in an aqueous medium results in the formation of hydroquinone and p-benzoquinone.
Additional preparative methods involve the acidic hydrolysis of nitrobenzene or p-nitrosophenol, carbonylation of acetylene, electrochemical oxidation of benzene or phenol in dilute sulfuric acid followed by reduction, and catalytic hydrogenation of nitrobenzene in acidic solution.
Furthermore, p-isopropenylphenol can be obtained through the alkaline cracking of bisphenol A and then reacted with 30% aqueous hydrogen peroxide under acidic conditions to produce hydroquinone.
2. Chemical Reactions of hydroquinone
Most oxidizing agents can easily convert hydroquinone to p-benzoquinone. Even neutral aqueous solutions of hydroquinone will darken upon exposure to air.
The rate of hydroquinone oxidation by air is accelerated in an alkaline solution. The oxidation product can react with water to form 1,2,4-benzenetriol, and further oxidation may lead to the formation of humic acids.
The redox potential E0 of hydroquinone is 699 mV, with a half-wave potential E1/2 of 560 mV at pH 0 and 234 mV at pH 5-6. The loss of an electron and the elimination of a proton during the oxidation of hydroquinone gives rise to a relatively stable semibenzoquinone radical, which enables hydroquinone to act as an antioxidant.
A second one-electron transfer and proton elimination leads to the formation of p-benzoquinone. The equimolar charge-transfer complex quinhydrone is formed by hydroquinone and p-benzoquinone, with the quinhydrone complex being typically dark greenish-black and having a melting point of 171°C.
Hydroquinone is a reducing agent with a reduction potential that is suitable for reducing silver halide. Grains of silver halide that have been exposed to light are reduced much faster than unexposed or underexposed grains, due to changes in the exposed silver halide crystals that reduce the energy barrier for reaction with hydroquinone to form elemental silver and p-benzoquinone.
Sodium sulfite is added to the developing solution to prevent the formation of quinhydrone by converting p-benzoquinone to sodium 2,5-dihydroxybenzenesulfonate, a weaker developing agent than hydroquinone.
The reactivity of hydroquinone is generally similar to that of phenol, with one or both hydroxyl groups being able to convert to an ether or ester.
Commercially important oxygen derivatives include hydroquinone monomethyl ether, hydroquinone dimethyl ether, and hydroquinone bis(2-hydroxyethyl) ether. Hydroquinone esters undergo the Fries rearrangement to give acyl-substituted hydroquinones.
Hydroquinone and its ethers can be C-alkylated under Friedel-Crafts conditions to produce a variety of mono- and disubstituted products, including 2-tert-butylhydroquinone, 2,5-bis[2-(2-methylbutyl)]hydroquinone, and 2-tert-butyl-4-methoxyphenol (BHA).
Hydroquinone also reacts with alkylamines or arylamines to form substituted arylamines, including p-N-methylaminophenol (usually marketed as the sulfate salt) and N,N’-diphenyl-p-phenylenediamine.
Hydroquinone can be chlorinated with chlorine or sulfuryl chloride to provide derivatives that range from mono- to tetrachlorinated.
The Kolbe-Schmitt carboxylation of hydroquinone with carbon dioxide leads to 2,5-dihydroxybenzoic acid (gentisic acid), while quinizarin (1,4-dihydroxyanthraquinone) can be prepared by the condensation of hydroquinone with phthalic anhydride.
Catalytic hydrogenation of hydroquinone gives 1,4-cyclohexanediol.
The sulfonation of hydroquinone results in the formation of hydroquinone mono- or disulfonic acid, typically isolated as the corresponding potassium salt.
3. Uses of hydroquinone
Hydroquinone and its derivatives are used in a variety of fields, including photography, rubber industry, dyes and pigments, antioxidants, agricultural chemicals, and other specialized applications.
Among these, the largest demand for hydroquinone arises from its use as a photographic developer, primarily in black-and-white film, lithography, photochemical machining, microfilm, and X-ray film.
Many hydroquinone derivatives, such as the sulfate salt of p-N-methylaminophenol and potassium 2,5-dihydroxybenzenesulfonate, are also employed in photographic applications.
The rubber industry is the second largest consumer of hydroquinone for the production of antioxidants and antiozonants. Notably, several hydroquinone derivatives, such as N,N’-diaryl-p-phenylenediamines, dialkylated hydroquinones, N-alkyl-p-aminophenols, dialkyl-p-phenylenediamines, and aralkyl-p-phenylenediamines, are used for this purpose.
Hydroquinone, hydroquinone monomethyl ether, and p-benzoquinone are extensively used in the vinyl monomer industry to inhibit free-radical polymerization during processing and storage.
Similarly, hydroquinone and several other derivatives, such as 2-tert-butylhydroquinone, 2-methylhydroquinone, and 2,5-di-tert-butylhydroquinone, serve as stabilizers for unsaturated polyester resins.
In addition, certain hydroquinone derivatives, including hydroquinone dimethyl ether, quinizarin, Chloroneb, and ethofumesate, are used in the manufacture of dyes, pigments, fungicides, and herbicides.
Fluazifop-butyl is an example of a new family of herbicides that use hydroquinone as a starting material.
Hydroquinone bis(2-hydroxyethyl) ether, formed by reacting hydroquinone with ethylene oxide, acts as a chain extender in thermosetting urethane polymers.
Hydroquinone and several derivatives, such as those of C-alkylated or C-arylated hydroquinone, are useful monomers for the preparation of a variety of polymers, including high-performance plastics, composites, and fibers.
Materials prepared using hydroquinone monomers exhibit high tensile and impact strengths, good weatherability, solvent resistance, flame retardance, transparency to microwave radiation, and retention of strength at elevated temperature.
Hydroquinone (U.S.P. grade) and several derivatives are used as skin bleaching and depigmenting agents in topical formulations.
Emerging applications for hydroquinone include its oxygen-scavenging properties that can be harnessed for use in boiler water treatment.
Reference
- Hydroquinone; Ullmann’s Encyclopedia of Industrial Chemistry. – https://onlinelibrary.wiley.com/doi/10.1002/14356007.a13_499