1. Friedel-Crafts Acylation
The Friedel-Crafts acylation involves the production of an aromatic ketone by the reaction between an aromatic compound and an acylating agent, which could be an acyl halide, an acid anhydride, an acid, or an ester. This reaction takes place in the presence of an acidic catalyst.
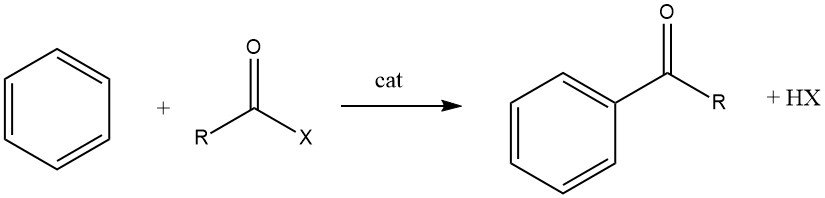
Table of Contents
The acylation of aromatic substrates holds significant industrial importance as it is utilized for the synthesis of aromatic intermediates extensively employed in the manufacturing of pharmaceuticals, insecticides, plasticizers, dyes, perfumes, and various other commercial products. These specialty compounds are typically produced on a smaller scale compared to alkylated products.
Acylating agent | Aromatic compound | Product | End use |
---|---|---|---|
Acetic anhydride | benzene | acetophenone | perfumes, pharmaceuticals, solvent, plasticizer |
Acetic anhydride | toluene | 4-methylacetophenone | perfumes |
Acetic anhydride | anisole | 4-methoxyacetophenone | perfumes |
Acetic anhydride | isobutylbenzene | 4-isobutylactophenone | pharmaceuticals |
Dichloroacetyl chloride | 1,2-dichlorobenzene | α,α,2,4-tetrachloroacetophenone | insecticides |
Chlorobutyroyl chloride | fluorobenzene | chloropropyl 4-fluorophenyl ketone | pharmaceuticals |
Tetrachloromethane | benzene | benzophenone | pharmaceuticals, insecticides, perfumes |
Benzoyl chloride | benzene | benzophenone | |
Phosgene | N,N-dimethylaniline | 4,4'-bis-dimethylaminobenzophenone | dyes |
Phthalic anhydride | benzene | 2-benzoylbenzoic acid | anthraquinone |
Acylation demonstrates remarkable versatility, giving rise to diverse products, including aromatic aldehydes, alkyl aryl ketones, symmetric and unsymmetric diaryl ketones, as well as cyclization products.
Acylating agents generally exhibit higher reactivity than alkylating agents, enabling acylations to be conducted under relatively mild conditions. Aromatic substrates with electron-donating substituents such as alkyl, hydroxyl, or alkoxy groups exhibit enhanced reactivity.
Conversely, aromatic compounds containing electron-withdrawing substituents like nitro, acyl, carboxyl, or nitrile groups are significantly more challenging to react, even with highly active acylating agents.
The introduction of the first acyl group inhibits the entry of a second acyl group, making disubstitution in the same ring rare. However, there are some exceptions, such as the diacylation of mesitylene, durene, and anisole using a mixture of glacial acetic acid and phosphorus pentoxide.
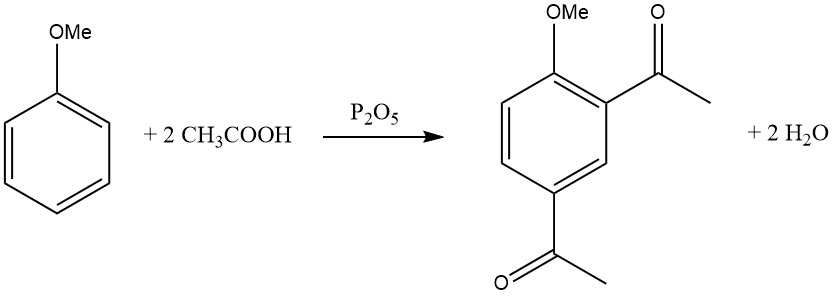
Electron-donating substituents facilitate acylation, and the reactivity enhancement due to polysubstitution is remarkable.
Apart from benzene and its derivatives, polynuclear carbocyclic aromatic compounds like naphthalene, diphenyl, anthracene, phenanthrene, and pyrene can also serve as substrates in acylation reactions.
Electron-rich heterocycles such as pyrrole, furan, and thiophene, along with their polycyclic derivatives, exhibit high reactivity.
In contrast, pyridine and quinoline do not react unless activated by substituents. Non-benzenoid aromatic substrates active in acylation include azulenes, ferrocenes, and indolizines.
Despite sharing some similarities with Friedel-Crafts alkylation, acylation displays characteristic differences.
For instance, consumption of the acidic catalyst is typically at least stoichiometric, though there are cases in the literature where substoichiometric amounts of Lewis acids are utilized. The reaction proceeds with higher regioselectivity and more uniformity compared to Friedel-Crafts alkylation, resulting in fewer isomerizations and side reactions.
1.1. Acylation Catalysts
The catalysts employed in Friedel-Crafts alkylation can also be used for acylation reactions. The catalyst of choice in most cases is AlCl3. Impurities present in AlCl3, such as FeCl3 or traces of water, enhance reaction yields and system reactivity, particularly in cases where reactions are otherwise slow.
However, in certain instances, the high activity of AlCl3 can lead to undesired side reactions, such as the decomposition of reactive heterocycles like benzofuran, ether decomposition, or alkyl group rearrangements.
To moderate the activity of AlCl3 in such cases, complexing solvents like nitrobenzene can be used to form 1:1 adducts. Additionally, BF3 and SnCl4 are suitable acylating catalysts for sensitive heterocycles.
When acylating agents such as anhydrides, esters, or carboxylic acids are employed, strong proton acids like sulfuric acid, perchloric acid, orthophosphoric acid, and polyphosphoric acid can also be applied.
Polyphosphoric acid, which was initially used mainly for intramolecular cyclization reactions, is now being increasingly used in intermolecular acylations.
The reaction of an acyl halide with an aromatic substrate, catalyzed by AlCl3, results in the formation of a stable complex of the Lewis acid with the aromatic ketone. The product is then liberated through hydrolysis.
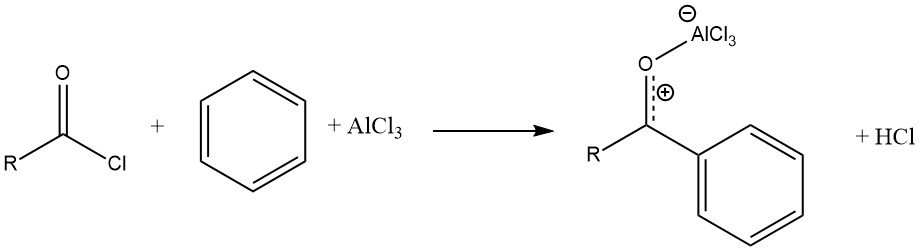
Unlike Friedel-Crafts alkylations, the catalyst must typically be applied in at least stoichiometric amounts with respect to the acyl halide. However, there are some exceptions where “truly catalytic” (substoichiometric) amounts of Lewis acids are used, such as ferrous chloride, zinc or iron oxides, cobalt chloride, and rare earth metal triflates.
For acylating agents like carboxylic acids or esters, at least twice the amount of catalyst is required, and in the case of anhydrides, three times the amount (but 1.5 mol per mole of product).
Hydrogen fluoride sometimes in combination with boron fluoride is an industrially important catalyst. It offers the advantage of catalyst recovery through distillation, thereby reducing waste formation.
This technology is commercially used for the acylation of isobutylbenzene to 4-isobutyacetophenone, an intermediate in the production of ibuprofen, a widely used anti-inflammatory drug.
1.1.1. Heterogeneous Catalysts
While the classical Friedel-Crafts synthesis with homogeneous catalysts remains the primary method for preparing aromatic ketones, it has several drawbacks:
1. It often requires stoichiometric or even excess amounts of “catalyst” due to the formation of stable complexes with the products. These catalysts, like AlCl3, act more like reagents than true catalysts, contributing significantly to production costs. They need to be separated and washed out of the product and cannot be easily recycled.
2. The reaction system is highly corrosive, necessitating expensive reactors and equipment for waste gas purification, which contains not only HCl but also chlorinated hydrocarbons.
3. The catalyst must be neutralized and disposed of along with a large volume of wastewater, adding to the environmental impact.
In recent years, efforts have been made to replace homogeneous catalysts with heterogeneous catalysts. Heterogeneous catalysts offer advantages such as not requiring stoichiometric amounts, being non-corrosive, easy separation without neutralization, and the possibility of recycling or using them in continuous fixed-bed processes.
Heterogeneous acylation catalysts need to be strong Lewis or Brønsted acids. Some important classes of solid acids that can catalyze this reaction are:
1. Zeolites: Microporous, crystalline alumo-silicates with strong Brønsted or Lewis centers. Medium-pore systems like H-ZSM-5 or large-pore systems like HY zeolite, beta zeolite, or mordenite have been successfully used for acylation reactions with electron-rich aromatic compounds.
2. Modified Clays: Clay minerals, when modified, can increase the number of acid sites. Acid-treated clays (Brønsted acid catalysts) and ion-exchanged clays (Lewis acid catalysts) are commercially used for various acid-catalyzed reactions.
3. Solid Superacids: Strong acids stronger than 100% sulfuric acid, including sulfated oxides like ZrO2, TiO2, HfO2, Fe2O3, and SnO2, have been studied as solid superacids for acylation reactions.
4. Miscellaneous: Other catalysts like heteropolyacids, surface-mounted acids (proton or Lewis acids on a support), and Nafion have also been investigated for acylation reactions, but they are not yet in commercial use.
By employing heterogeneous catalysts, researchers aim to overcome the limitations of homogeneous catalysts and develop more sustainable and efficient processes for aromatic ketone synthesis.
1.2. Solvents in acylation reaction
For acylation reactions using an acyl halide – Lewis acid catalyst system, the choice of solvent plays a crucial role. Non-polar solvents like CCl4 or CS2, as well as solvents of medium polarity like dichloromethane or 1,2-dichloroethane, can be used as the aromatic substrate.
With AlCl3 as the catalyst, heterogeneous systems are formed in these solvents. However, using solvents like nitromethane or nitrobenzene results in homogeneous solutions and reduced reactivity due to complex formation. The choice of solvent can also influence the regioselectivity of the acylation reaction.
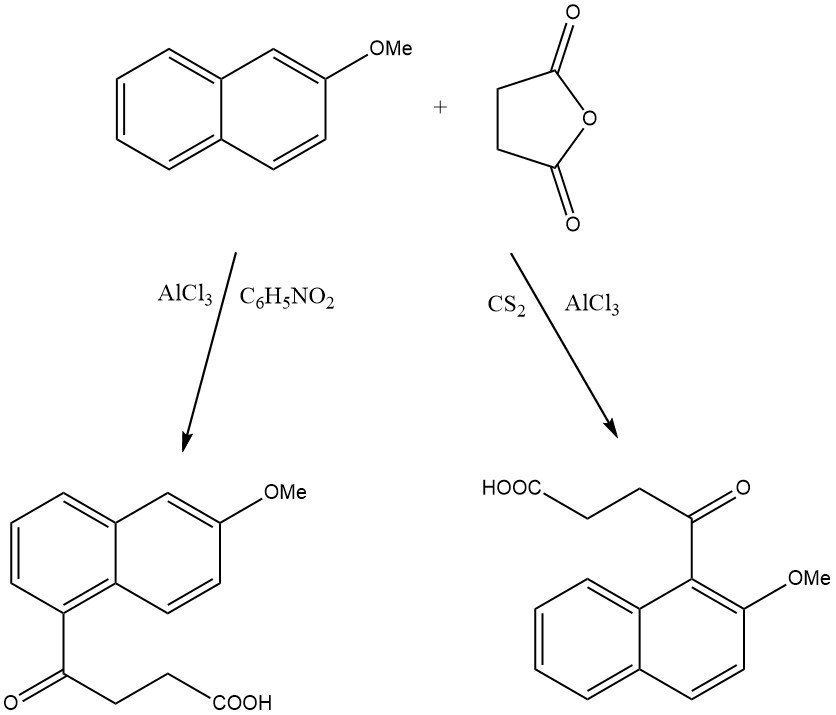
One hazard to consider is that adducts of AlCl3 and nitrobenzene can decompose explosively at elevated temperatures.
There are three common procedures for carrying out acylation using an acyl halide – Lewis acid catalyst system:
1. The acylating agent is added to a cooled solution or suspension of the catalyst in a solvent, followed by the addition of the aromatic substrate to the preformed acylating complex (Perrier method).
2. All reactants are mixed and cooled, and the catalyst is slowly added (Elbs method).
3. The aromatic substrate acts as the solvent for the catalyst, and the acylating agent is then slowly added (Bouveault method).
The Perrier method is often preferred as it maintains a constant ratio of catalyst to acylating agent throughout the reaction.
If sulfuric acid is used as the catalyst, a large excess of the acid is required, and the reactants are added to it. To avoid ring sulfonation or aldolization reactions, the temperature should be kept below 100 °C.
1.3. Acylating Agents
Friedel-Crafts acylation exhibits remarkable versatility due to the systematic variation that can be introduced in both the acylating agent and the aromatic substrate. The reactivity of acylating agents follows a trend as follows:
[RCO]+[BF4]− > [RCO]+[ClO4]− > RCOO-SO3H > RCOX (RCO)2 O > RCO2R’ > RCONR’2
Ionic acyl perchlorates and tetrafluoroborates are potent agents, whereas acid esters and amides are nearly inactive without catalysts. The reactivity of acyl halides decreases in the order: RCOI > RCOBr > RCOCl > RCOF.
The reactivity of acyl halides is also influenced by the substituent R, which can be alkyl or aryl and may contain halogen, alkyl, alkoxy, or nitro groups. The reactivity is further influenced by the type of aromatic substrate and the catalyst used.
Aromatic acyl halides can contain nitro substituents or be of the pyridine type, as demonstrated in the synthesis of 3-benzoylpyridine from benzene and nicotinyl chloride.
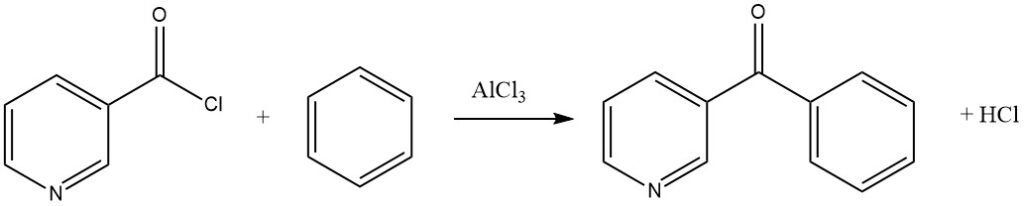
Bifunctional acyl halides can also act as acylating agents, as seen in the reaction of oxalyl chloride with an activated aromatic substrate like anisole, leading to the corresponding benzil derivative.
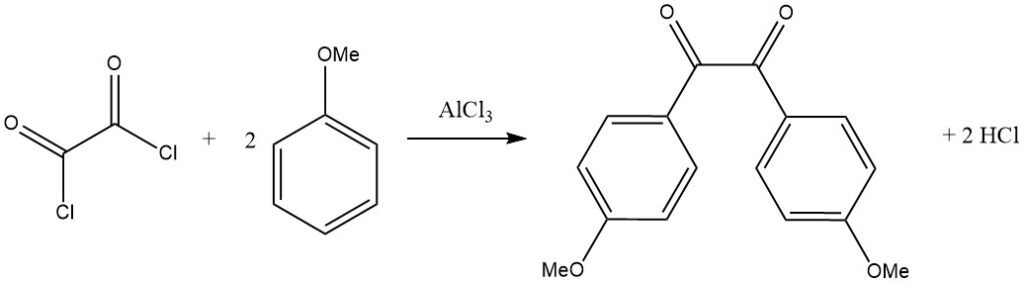
Phosgene, a commercially important reagent, can react with N,N-dimethylaniline to produce 4,4′-bis(dimethylamino)benzophenone (Michler’s ketone), used in dye production.
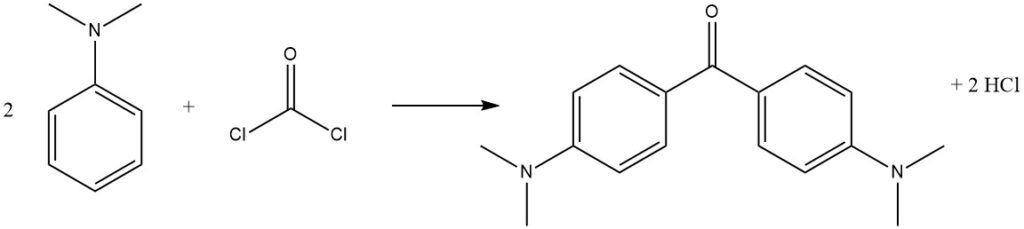
By replacing one halogen of phosgene with an amido group, the resulting amide can be hydrolyzed to form the acid, which is considered for the industrial synthesis of terephthalic acid.
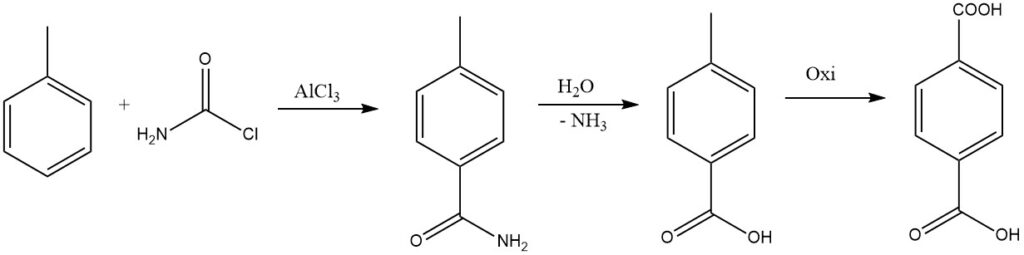
Isocyanates, isothiocyanates, and CO2 can also serve as acylating agents in the presence of AlCl3 to yield aromatic carboxylic acids or their derivatives.
Unsymmetrical bifunctional acylating agents, such as acid chlorides containing an ester group, selectively react with the more active acyl group.
Similarly, acid anhydrides, such as succinic, glutaric, or maleic anhydrides, in the presence of 2 moles of AlCl3 per mole of anhydride, can produce 4-aryl-4-oxobutanoic acids, which are intermediates in the Haworth synthesis of polynuclear aromatics.
The synthesis of anthraquinone from phthalic anhydride and benzene is of commercial significance. Under strongly acidic conditions, 3,3-diphenylphthalide can also form.

With zeolite catalysts, non-activated carboxylic acids can be used in the liquid phase to react with toluene, yielding p-acyltoluenes with high yields. This process has shown great promise, with yields reaching up to 96%.
2. Acylation of Benzene and Benzene Derivatives
2.1. Acylation of Benzene
The industrial acylation of benzene with acetic anhydride, using AlCl3 as the catalyst and maintaining a temperature of 30 °C through cooling, results in acetophenone with an 85% yield. However, acetophenone is now predominantly produced industrially by the oxidation of ethylbenzene or cumene.
Another method involves the gas-phase acylation of benzene with benzoic acid at elevated temperatures, yielding benzophenone.
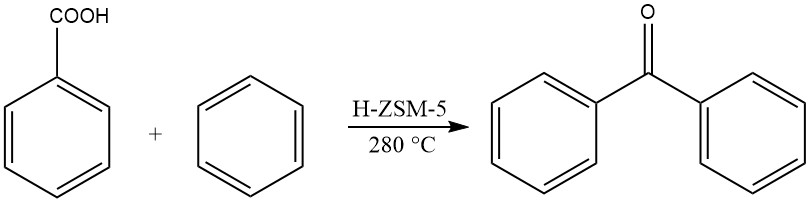
2.2. Acylation of Toluene
Acylation of toluene, using acetyl chloride and aluminum chloride as the catalyst, results in the formation of 4-methylacetophenone with an impressive selectivity of 97.6%. This compound finds application in the production of perfumes.
2.3. Acylation of Deactivated Substrates
Benzenoid aromatic compounds containing nitro, carboxyl, nitrile, and acyl substituents exhibit hindrance to electrophilic substitution. However, acylation of these compounds becomes feasible only in the presence of an activating second substituent, such as a hydroxy or alkoxy group.
On the other hand, deactivated substrates like chlorobenzene can be subjected to acylation to form p-chlorobenzophenones, utilizing commercial heterogeneous catalysts like zeolites or sulfated zirconia, known for their properties as solid superacids.
2.4. Acylation of Activated Substrates
The acylation of activated substrates, such as phenol, can be accomplished using various catalysts, including carboxylic acid – ZnCl2, carboxylic acid – BF3, or carboxylic acid – polyphosphorus acid. The acylation of phenols demonstrates up to 25% selectivity for ortho substitution, with the ortho to para ratio being strongly influenced by the choice of catalysts and solvents.
Linear alkylphenols can be synthesized through acylation followed by reduction. An illustrative example is the production of hexylresorcinol, widely used as a disinfectant:
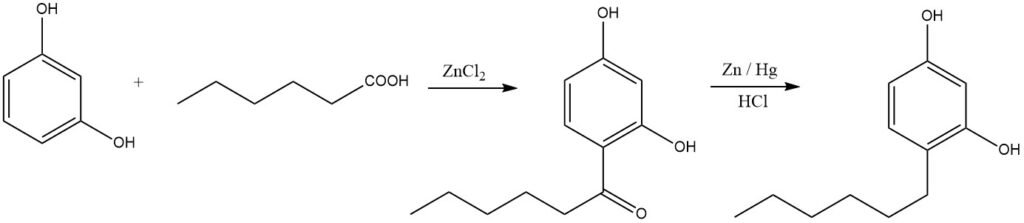
Both phenols and phenyl ethers are highly reactive substrates and exhibit reactivity under relatively mild conditions. For instance, anisole can be conveniently acylated at 40 – 50 °C in the presence of polyphosphoric acid. Phenyl ethers, on the other hand, undergo acylation predominantly in the 4 position.
An environmentally friendly alternative to conventional Friedel – Craft acylation systems avoids the formation of waste salts and byproducts. This approach involves the use of mixed anhydrides of trifluoroacetic acid, which are generated in situ by reacting with carboxylic acids. Low concentrations of H3PO4 serve as the catalyst, and trifluoroacetic acid can be recycled after dehydration.
2.5. Acylation of Aromatic Amines
Following N-acylation, aromatic amines exhibit a reactivity pattern akin to alkylaromatic compounds in acylation reactions. Tertiary aromatic amines, such as N,N-dimethylaniline, can undergo acylation at the 4 position, employing P2O5 as the catalyst. This is well known in the synthesis of Michler’s ketone.
2.6. Cyclization Reactions
6-methoxy-1-tetralone is obtained in an impressive 96% yield by reacting 4-(3-methoxyphenyl)butyric acid.
Intermolecular cyclizations are prominently demonstrated in reactions of phthalic anhydride with benzene and its derivatives, resulting in anthraquinone and substituted anthraquinones, respectively.
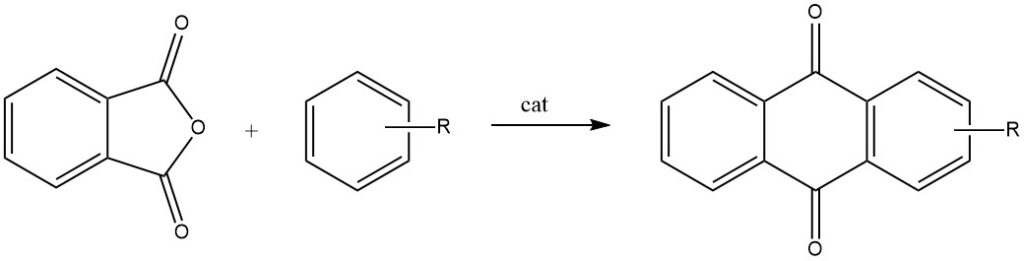
When phthalic anhydride reacts with toluene or chlorobenzene, it undergoes a two-step process, leading to the formation of 2-substituted anthraquinones.
Conversely, the reaction with 4-chlorophenol directly yields 1,4-dihydroxyanthraquinone (chinizarine). Subsequent oxidation, nitration, chlorination, or amination of these products generates essential intermediates for fine chemicals.
Moreover, the Friedel – Crafts acylation of ethylbenzene with phthalic anhydride produces, through a two-step process, 2-ethylanthraquinone, a significant component utilized in the synthesis of H2O2.
3. Acylation of Polynuclear Aromatic Compounds
3.1. Acylation of Naphthalene
Acylation of naphthalene typically results in mixtures of 1- and 2-acyl derivatives, and the relative proportions of these derivatives are mainly influenced by the choice of solvent. When non-polar solvents like CS2 or CCl4 are used, acylation primarily occurs in the 1 position. Conversely, in polar solvents such as CH3NO2 or C6H5NO2, the formation of 2-acetylnaphthalenes is favored.
3.2. Acylation of Substituted Naphthalenes
The acylation of substituted naphthalenes often yields complex mixtures of products. Typically, the more highly activated ring is more prone to acylation. However, the regioselectivity can be controlled by the shape selectivity of zeolites.
For instance, when 2-methoxynaphthalene is acylated on zeolite HY, only 1-acetyl-2-methoxynaphthalene is produced, while on beta zeolite, 2-acetyl-6-methoxynaphthalene is formed.
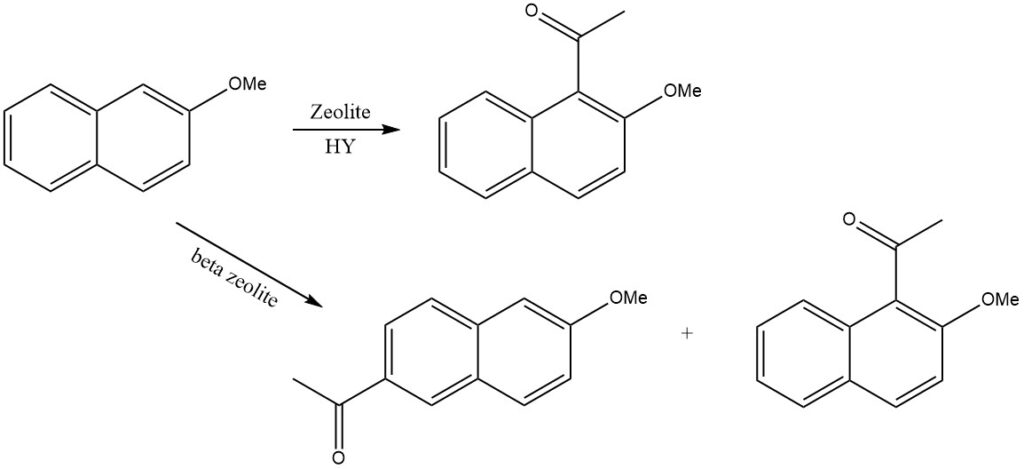
1,5-diacylation requires more stringent conditions, as seen in the synthesis of 1,5-dibenzoylnaphthalene. This compound can be further fused in sodium aluminum chloride to yield dibenzopyrenequinone, a dye commercialized as Indanthren Goldgelb GK.
When naphthalene reacts with diacyl compounds, the 1,8-diacylated products are often formed.
3.3. Acylation of Biphenyls
When one ring of a biphenyl molecule is deactivated by an electron-withdrawing substituent, the other ring becomes more reactive and can undergo acylation at the 4 position. The deactivation of one ring enhances the reactivity of the other ring, making it more susceptible to electrophilic substitution, and thus, acylation occurs preferentially at the 4 position.
3.4. Acylation of Anthracene
In the presence of AlCl3 and under mild conditions in nonpolar solvents (0 °C, benzene), anthracene undergoes acylation at the most reactive 9 position. Isomerization of the ketone – AlCl3 complex is prevented by precipitation.
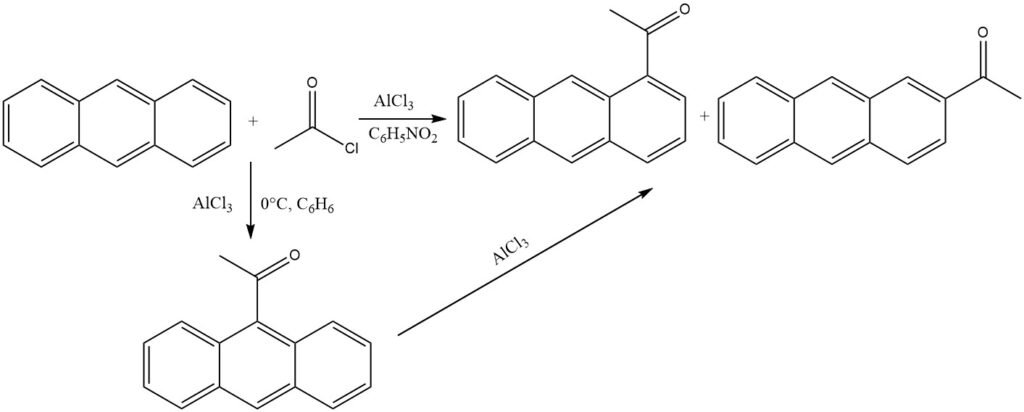
However, when polar solvents like nitrobenzene are used, a mixture primarily composed of 1-acylanthracene and some 2-acylanthracene is formed. In this scenario as well, 9-acylanthracene is likely the initial product, which subsequently undergoes isomerization.
3.5. Acylation of Phenanthrene
Likewise, when phenanthrene is acylated in nitrobenzene, the main products obtained are the 2- and 3-acyl isomers. On the other hand, using the less polar solvent dichloroethane predominantly leads to the formation of the 9-acyl isomer as the primary product. The choice of solvent plays a crucial role in determining the regioselectivity of the acylation reaction for phenanthrene.
4. Acylation of Heteroaromatic Compounds
Furan, thiophene, and pyrrole possess high electron density, rendering them highly activated in electrophilic substitution reactions, allowing for their acylation under mild reaction conditions.
4.1. Acylation of Furan
Acylation of furan results in significant yields of the 2-acyl derivative, particularly when anhydrides serve as the acylating agents. The excellent reactivity of furan permits the use of benzene as a solvent. Suitable catalysts for this reaction include zinc chloride, boron trifluoride, and phosphoric acid. Additionally, acylation can also take place at the 5 position.

4.2. Acylation of Thiophene
The reactivity of thiophene can be likened to that of anisole. Thiophene can be efficiently acylated, yielding high yields of the desired products, when acyl halides are used in the presence of SnCl4 or anhydrides are employed with phosphoric acid or ZnCl2 as the acylating agent.
4.3. Acylation of Pyrrole
Acylation of pyrrole can take place even in the absence of catalysts, although ZnCl2, BF3, and AlCl3 are commonly used in conjunction with anhydrides as acylating agents. Generally, acylation occurs at the 2 position of the pyrrole ring, but 2,5-diacylation is also feasible. However, 3-acylation is observed only when both the 2 and 5 positions on the pyrrole ring are blocked.
4.4. Acylation of Imidazoles
Heterocycles that are highly deactivated and cannot be acylated in homogeneous liquid phases, such as imidazole or pyrazoles, are capable of reacting on Na-Y-zeolites at elevated temperatures exceeding 500 °C.

4.5. Acylation of Benzofurans
Highly reactive heterocycles can undergo acylations using zeolites in a continuous liquid-phase process. For instance, the acylation of 2-methylbenzofuran with acetic anhydride is a representative example:
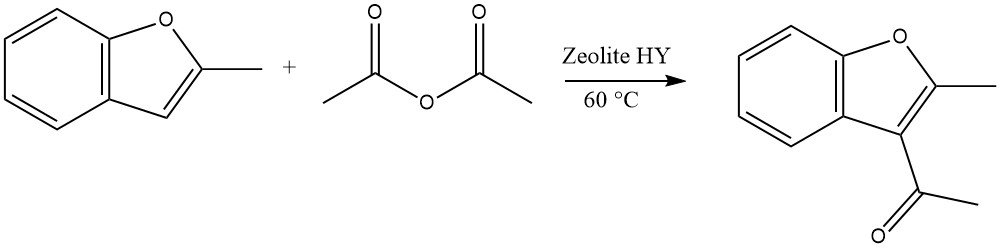
In the case of dibenzofuran and dibenzothiophene, acylation or diacylation can occur at the 2 or 8 position. For carbazole, acylation or diacylation typically occurs at the 3 or 6 position.
4.6. Miscellaneous
Substituted 4H-pyrones have demonstrated excellent yields in acylation reactions when trifluoroacetic acid is used as the catalyst. Notably, even diacylation reactions are achievable.

A wide range of other heteroaromatic compounds, including chroman, xanthenes, 1,3-benzodioxole, and dibenzodioxins, have also been successfully subjected to acylation reactions.
5. Acylation of Nonbenzenoid Aromatic Compounds
Ferrocene readily undergoes acylation with acyl halides in the presence of aluminum chloride, displaying comparable reactivity to phenols. Anhydrides can also serve as acylating agents. With BF3 as the catalyst, monoacylation of ferrocene can be achieved. When an excess of both acylating agent and AlCl3 is used, heteroannular diacetylation becomes the predominant reaction.
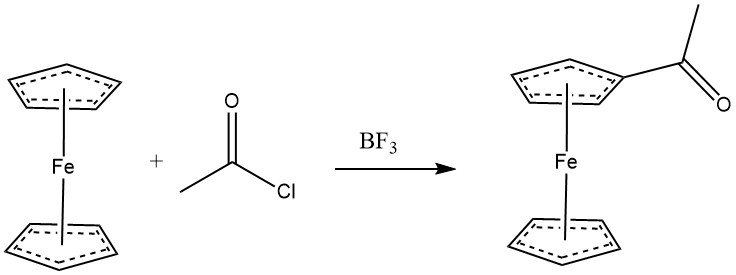
Various cyclopentadienyl derivatives are amenable to acylation. The reactivity of some aromatic substrates follows this order: C6H5OH•(C5H5)2Fe > C6H5OCH3 > CH3C5H4Mn(CO)3 > C5H5Mn(CO)3 > C6H6.
Azulene smoothly reacts with acetic anhydride in the presence of SnCl4 as a catalyst, leading to the acylation of the cyclopentadienyl part and resulting in 1-acetylazulene. Under more rigorous conditions and in the presence of AlCl3 or SnCl4, 1,3-diacetylazulene is formed by using acetyl chloride or acetic anhydride as the acylating agent.
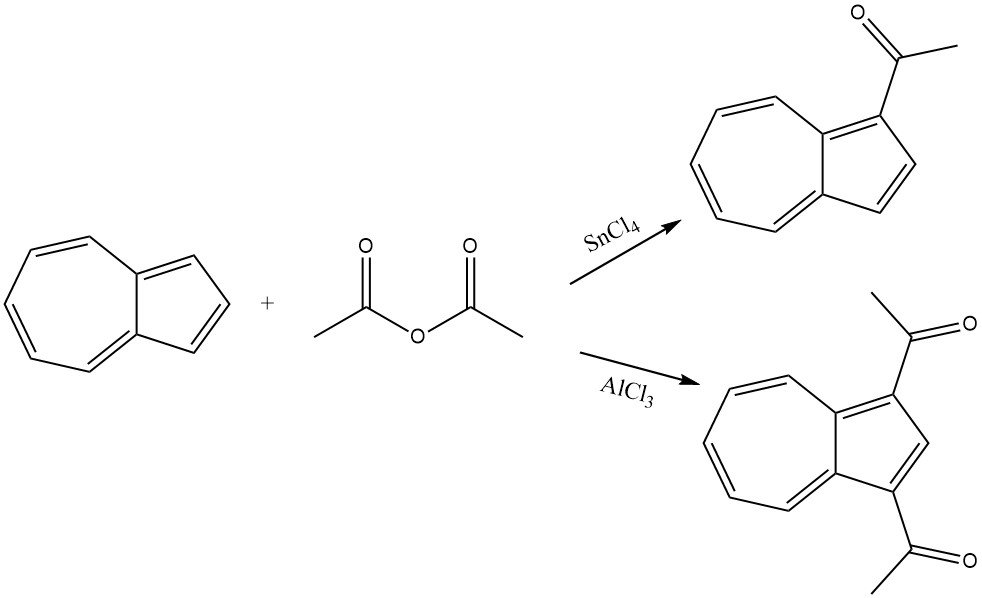
6. Miscellaneous Acylation Reactions
Among the numerous reactions leading to aromatic C-acylation products, the formylation reactions, the Houben-Hoesch synthesis, and the Fries rearrangement are particularly significant.
6.1. Formylation
Formylation of aromatic compounds is a specific type of acylation reaction that occurs when a formyl halide or formic anhydride reacts with an aromatic compound in the presence of a Friedel-Crafts catalyst, such as AlCl3. However, the application of this reaction is limited due to the instability of most formylating agents.
The Gattermann-Koch synthesis uses a mixture of hydrogen chloride and carbon monoxide with AlCl3 and copper (I) chloride as the formylating agent for aromatic compounds. Benzene, as well as substituted benzenes or polycyclic aromatics, undergo formylation to yield aromatic aldehydes, with a preference for the para isomers.
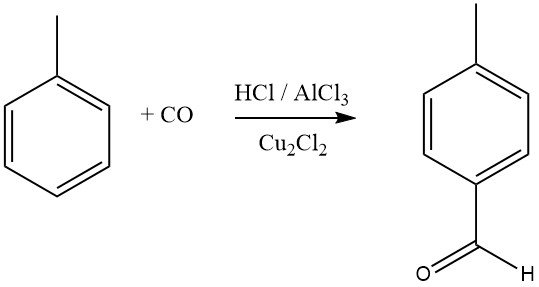
Aromatic compounds with meta-directing substituents do not react in this formylation process. Mitsubishi Gas Chemical is exploring a modified Gattermann-Koch synthesis for the industrial synthesis of terephthalic acid.
In this process, toluene reacts with CO and HF-BF3 to produce p-tolualdehyde in high yield, which is then oxidized to terephthalic acid. Recent studies suggest that the actual acylating agent in these reactions is [CHO]+, formed by protonation of CO or its complexes.
For the Vilsmeier aldehyde synthesis, aromatic and heterocyclic substrates are formylated by reacting with dialkyl- or arylalkylformamides in the presence of POCl3. This reaction allows formylation of a variety of compounds, including reactive polynuclear aromatic compounds, phenol ethers, dialkylamines, and N-heterocycles like pyrrole and indole.
The regioselectivity of formylation follows the principles of Friedel-Crafts reactions, typically leading to the formation of 4-substituted aromatic compounds. Additionally, aromatic substrates can be formylated using dichloromethyl methyl ether or trialkyl orthoformate in the presence of TiCl4 or AlCl3, respectively.
6.2. Houben-Hoesch Synthesis
The Houben-Hoesch synthesis, closely related to the Gattermann reaction, involves the acylation of reactive aromatic substrates like phenols, phenyl ethers, pyrroles, thiophenes, and indoles using nitriles. These reactions take place in the presence of hydrogen chloride (HCl) and zinc chloride (ZnCl2).
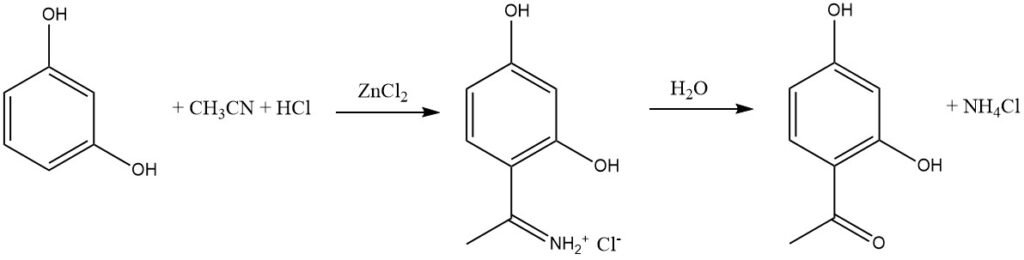
In this synthesis, the active electrophile is the imminium ion, which is formed by the addition of HCl to the nitrile and then complexed by the Lewis acid (ZnCl2). This bulky intermediate predominantly leads to acylation at the 4 position of the aromatic substrate rather than the 2 position.
When compared to Friedel-Crafts acylation with acyl halides or anhydrides, the Houben-Hoesch synthesis is often a more straightforward method for acylating activated phenols.
6.3. Fries Rearrangement
The Fries rearrangement of aryl esters, which can be prepared through the acylation of phenols, is a valuable method for synthesizing phenolic ketones used in the production of fine chemicals. This reaction is extensively employed in the pharmaceutical industry for the synthesis of drugs like adrenalon, synephrin, buphenin, and acebutolol.
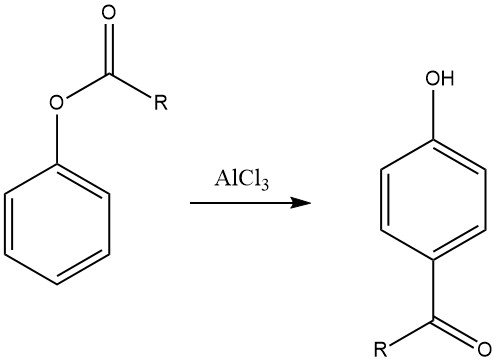
The regioselectivity of the Fries rearrangement can be controlled by adjusting reaction conditions. Generally, para substitution is favored at low temperatures, while ortho substitution is favored at high temperatures. However, the choice of solvent and the type and quantity of catalysts also influence the regioselectivity.
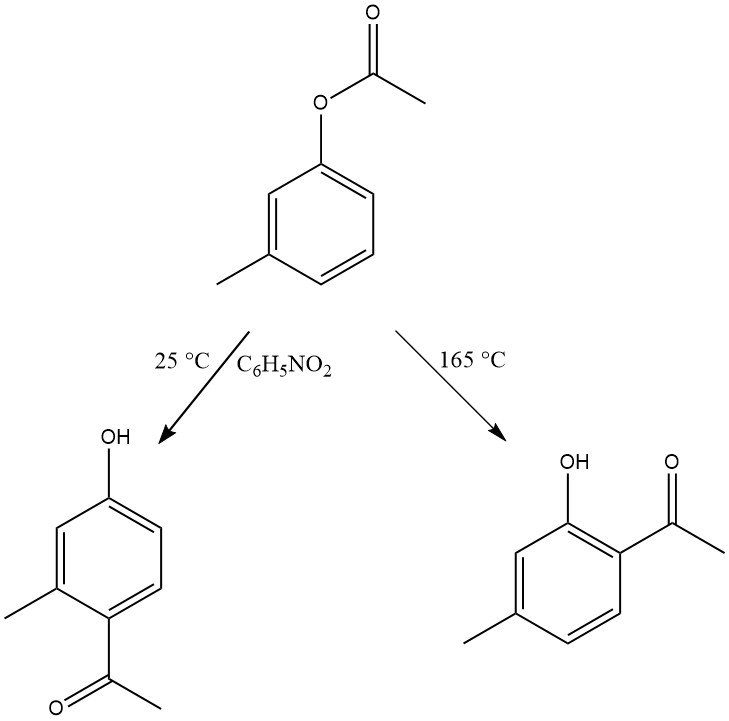
A broad range of substrates with various substitutions in both the phenol and acid moieties can undergo this rearrangement. Even phenol derivatives with electron-withdrawing groups, like the nitro group, as well as naphthol and hydroxy- or dihydroxyphenyl esters, are reactive in this transformation.
N-Acylanilines can also be rearranged to yield 4-acylanilines under elevated temperatures. The acid part of the esters can be varied, allowing the use of different alkyl or aryl acids, including diaryl dicarboxyl esters and sulfonic acid esters, which lead to the formation of 4-hydroxyphenyl sulfones.
AlCl3 is the commonly used catalyst, although SnCl4, TiCl4, FeCl3, and ZnCl2 are also effective and sometimes more convenient. The rearrangement is typically performed by heating a mixture of phenolic esters and the catalyst to temperatures ranging from 80 to 180 °C.
Depending on the substrate’s reactivity, the reaction can be completed within a few minutes to a few hours. It is possible to conduct the reaction at lower temperatures in solvents like C6H6NO2, (CH2Cl)2, CS2, C6H5Cl, or petroleum ether, but this requires longer reaction times.
Alternatively, the rearrangement can be initiated in CS2, followed by distillation to remove the solvent, and then completed at higher temperatures.
To isolate the ortho isomer from the product mixtures, steam distillation is often employed. On the other hand, volatile products can be separated by fractional distillation, and the para isomer can be obtained from nonvolatile product mixtures through crystallization.
Reference
- Acylation and Alkylation; Ullmann’s Encyclopedia of Industrial Chemistry. – https://onlinelibrary.wiley.com/doi/10.1002/14356007.a01_185
FAQ about Acylation
Friedel-Crafts acylation is a type of organic chemical reaction that involves the introduction of an acyl group (R-CO-) onto an aromatic ring. It is named after the chemists Charles Friedel and James Crafts, who developed the reaction in the 19th century.
Acidification of the reaction mixture before acylation is often necessary to generate an appropriate electrophile that can react with the aromatic substrate. This step helps in the activation of the acylating agent (e.g., acyl halide or anhydride) and prepares it to undergo electrophilic aromatic substitution, a key step in Friedel-Crafts acylation. The acidification process involves the addition of a Lewis acid catalyst like AlCl3 or FeCl3 to generate the reactive acylium ion (RCO+), which facilitates the acylation reaction.
Friedel-Crafts acylation involves the electrophilic substitution of an acyl group onto an aromatic ring. The reaction takes place by first generating a strong electrophile (acylium ion) from the acylating agent in the presence of a Lewis acid catalyst. This electrophile then attacks the electron-rich aromatic ring, leading to the formation of an intermediate carbocation. The reaction is completed by the deprotonation of this carbocation, which results in the introduction of the acyl group on the aromatic ring, producing the acylated product.
Acylation reactions can be performed in a variety of solvents depending on the specific reaction conditions and the reactivity of the substrates and reagents involved. Non-polar solvents such as CCl4, CS2, benzene, and toluene are commonly used for Friedel-Crafts acylation reactions. Additionally, polar solvents like dichloromethane and nitrobenzene can be employed for specific reactions. The choice of solvent can influence the reactivity, regioselectivity, and yield of the acylation reaction.
The strong desactivating effect of the acyl group leads to the formation of monosubstitution products predominantly and the bulky nature of the acylating group also restricts further substitution, leading to monosubstitution as the major product.
Acylation reactions have broad applications in organic synthesis and the production of various chemical compounds. Some common uses of acylation include:
– Synthesis of aromatic ketones, essential intermediates in pharmaceuticals and agrochemicals.
– Preparation of fine chemicals, dyes, and perfumes.
– Formation of reactive intermediates for further functionalization of organic molecules.
– Production of industrial chemicals like terephthalic acid and anthraquinones.
– Synthesis of biologically active compounds, such as drugs and natural products.
While Friedel-Crafts acylation is a valuable synthetic method, it has some limitations. One significant limitation is the potential for side reactions, such as polyacylation, which can lead to undesirable products and decrease the overall yield. Additionally, some substrates may not undergo acylation efficiently due to steric hindrance or deactivation caused by certain substituents. Moreover, the use of strong Lewis acid catalysts can lead to undesired side reactions or catalyst poisoning.
Yes, Friedel-Crafts acylation has been widely utilized in the synthesis of various natural products and biologically active compounds. It allows the introduction of specific functional groups into aromatic rings, facilitating the creation of diverse chemical structures found in natural products. The regioselectivity and mild reaction conditions make it a valuable tool for the efficient synthesis of complex molecules with aromatic moieties.
Friedel-Crafts acylation reactions often involve the use of strong Lewis acids and acylating agents, which can be hazardous and reactive. Proper safety precautions should be followed, including the use of appropriate protective equipment, such as gloves, lab coats, and safety goggles. The reactions should be conducted in a well-ventilated fume hood to avoid inhalation of acid vapors.