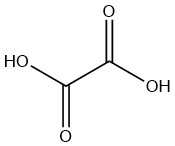
Oxalic acid is the simplest saturated dicarboxylic acid with the formula C2H2O4. It is a white solid that occurs naturally in the form of a dihydrate. Anhydrous compound must be produced from the dihydrate even when manufactured industrially.
Oxalic acid is found naturally in many plants, including leafy greens, vegetables, fruits, cocoa, nuts, and seeds. It’s also found in rhubarb leaves and certain types of beans.
It has been known for a long time and was first identified by WIEGLEB in 1769 in the potassium salt of sorrel.
Table of Contents
1. Production of Oxalic acid
There are numerous industrial processes used in the production of oxalic acid, which have been implemented by various companies and are still in use today.
In general, only three types of compounds are used as raw materials in the production of oxalic acid. These include carbohydrates (such as molasses and ethylene glycol), olefins, and carbon monoxide.
In all industrial processes, oxalic acid is obtained as the crystalline dihydrate. The following sections describe four methods that are currently used industrially. Three of these methods are based on nitric acid oxidation, and one is a synthesis that uses carbon monoxide.
1.1. Carbohydrate Oxidation
This method, which is the oldest of the chemical productions, was first discovered by SCHEELE more than 200 years ago in 1776. He oxidized sugar with concentrated nitric acid to produce oxalic acid.
However, it was not until around 1940 that this process gained industrial importance, when the nitrogen oxides produced in the reaction could be recovered and recycled.
In Germany, I.G. Farben produced 2000 tonnes of oxalic acid per year using this method until 1944. This process is currently used in Brazil, China, and in several Eastern European countries.
The raw materials used in this process include sugar, glucose, fructose, corn starch, wheat starch, reclaimed starch, potato starch, corncobs, tapioca, and molasses.

The selection of starting materials for the production of oxalic acid depends on availability, cost, and the optimization of the reaction process.
Different raw materials can result in varying yields and the formation of foam, greases, and slime, which need to be managed appropriately.
Molasses and other agricultural waste have become increasingly popular as raw materials due to their low cost and the environmental benefits of their utilization.
Molasses, which is a byproduct of sugar refinement, contains nitrogen-containing compounds that are mostly eliminated during nitric acid oxidation, despite causing excessive foam formation during the reaction.
In the case of using starch-based materials as raw materials, they must first be hydrolyzed to glucose in a separate batchwise step prior to oxidation.
The hydrolyzed glucose solution is mixed with sulfuric acid, vanadium pentoxide (0.001-0.05 wt%), and iron (III) sulfate (0.39-0.8 wt%) in a reactor.
Nitric acid (65%) is then added slowly with vigorous stirring within a tightly controlled temperature range around 70°C, varying based on the raw material.
The reaction is strongly exothermic and must be well-cooled.
Air is simultaneously supplied to the reactor to support the reaction and to remove NO and other nitrogen oxides of low oxidation number. The expelled nitrogen oxides are collected in an adsorption system and recycled after oxidation.
The crude oxalic acid is obtained by cooling and centrifugation of the reaction mixture, followed by dissolution in hot water, grease separation, recrystallization, a second centrifugation, and drying to obtain oxalic acid dihydrate with purity greater than 99%.
1.2. Oxidation of Ethylene Glycol
The oxidation of ethylene glycol with nitric acid is a one-step process. An oxidizing mixture of 30-40% sulfuric acid and 20-25% nitric acid is used, and the reaction is carried out at 50-70°C and atmospheric pressure in the presence of vanadium pentoxide and iron (III) salts. The byproduct formed during this reaction is only CO2.
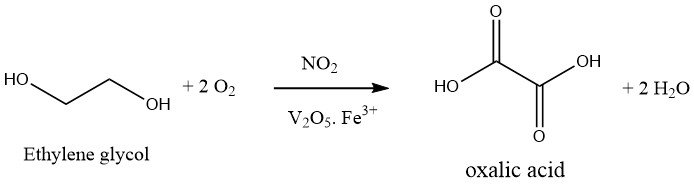
The process of oxalic acid production has undergone significant enhancements by Mitsubishi Gas Company, resulting in Japan’s primary reliance on this method.
The improved technique entails the use of a pressure reactor operating at around 10 bar. Nitric acid (approximately 60%), as well as sulfuric acid (where applicable), are subjected to pressurized oxygen, while maintaining a temperature of 80°C.
Subsequently, ethylene glycol is introduced continuously, and the temperature and pressure of the reaction are maintained via the addition of oxygen. Effective cooling is required to prevent the exothermic reaction from rising above 50-70°C.
The output yield of oxalic acid, based on ethylene glycol, ranges between 90-94% of the theoretical maximum. Notably, the majority of the nitric acid utilized can be recovered. This suggests that nitrogen oxides and nitric acid are merely acting as catalysts in the oxidation process, with oxygen serving as the primary oxidizing agent.
Alternative starting materials, such as propylene glycol, acetaldehyde, or glycolic acid, can also be used. To improve efficiency, vanadium compounds (0.001 – 0.1 wt %) are recommended as promoters. Initiators such as sodium nitrite, formic acid, or formaldehyde may also be employed.
1.3. Oxidation of Propene
The production of oxalic acid through propene and nitric acid reaction is the second most significant industrial process after carbohydrate oxidation.
In 1978, this method accounted for 65,000 t/a of global oxalic acid production. Petrochemical refinery by-products, namely pure propene or propene-rich fractions, serve as feasible feedstocks for this reaction.
Rhone-Poulenc, a French-based corporation, significantly improved the process by introducing technical engineering and construction advancements while maintaining strict adherence to reaction conditions.
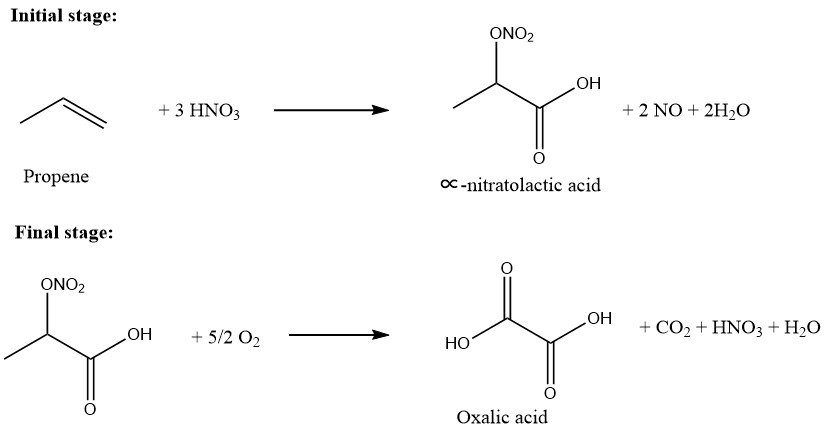
The process of propene oxidation to oxalic acid comprises two primary stages.
In the initial step, propene is introduced into a nitric acid solution (50-75 wt %) at a temperature range of 10-40°C. The molar ratio of propene to nitric acid is maintained between 0.01/1.0 and 0.5/1.0. This step yields water-soluble ∝-nitratolactic acid and lactic acid intermediates.
In the second stage, oxygen is introduced into the solution containing the partially oxidized products in the presence of a catalyst, at a temperature range of 45-100°C.
The resultant oxalic acid is subsequently crystallized, filtered, and recovered. The overall yield, based on propene, is approximately 90%, and the conversion of propene is almost 100%.
Nitrogen oxides generated in the second reactor are eliminated and recovered. The consumption of nitric acid in this process is 1.2 kg per kg of oxalic acid dihydrate produced.
Catalysts containing salts or compounds of Fe, Al, Cr, Sn, Bi, or I are added to improve the yield. In this process, chromium (III) nitrate, iron (III) nitrate, or tin (II) chloride are utilized in quantities of 0.4-0.5 wt% (based on the metal) and dissolved in 65% nitric acid to enhance efficiency.
1.4. Production from Carbon Monoxide
Ube Industries has developed the most recent process for the industrial production of oxalic acid, which involves two steps. In the first stage, CO and a lower alcohol are reacted under pressure and in the presence of a catalyst to form the corresponding diester of oxalic acid.
In the second step, hydrolysis of this diester results in the formation of oxalic acid and the original alcohol, which is recycled. The reaction is catalyzed by palladium on charcoal and alkyl nitrites, and carried out at a pressure of 10-11 MPa. The preferred alcohol and solvent is n-butanol.
While this process benefits from the use of inexpensive starting materials, it has significant drawbacks due to the much higher capital investment (due to high-pressure requirements) and utility costs.
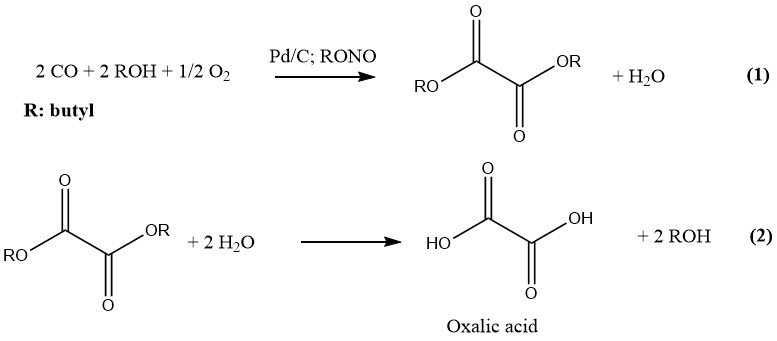
Butyl nitrite and methyl nitrite are the preferred alkyl nitrites for industrial purposes due to their suitability in the production of oxalic acid. A plant constructed in 1978 employed butyl nitrite in the production of oxalic acid at a rate of 6000 t/a.
Butyl nitrite offers several advantages in this process, including its function as both a reaction component and a dehydrating agent. The higher boiling point of butyl nitrite compared to water and butyl nitrite allows for the removal of water formed as an azeotropic mixture, leaving most of the butyl nitrite in the system.
The recovered butyl nitrite can be reused after separation. Butyl nitrite is finally hydrolyzed to butanol, which can be easily separated from the oxalic acid solution by phase separation.
The reaction for the production of oxalic acid using butyl nitrite and a palladium catalyst takes place in a reaction tower. A circulation liquid containing butyl nitrite, butanol, and the palladium catalyst, as well as a circulation gas containing carbon monoxide and oxygen, are pressurized and fed to the tower.
The exothermic reaction between butyl nitrite and carbon monoxide results in the formation of dibutyl oxalate and the simultaneous regeneration of butyl nitrite. The reaction is conducted at a temperature of 90 – 100 °C and a pressure of 10 –11 MPa in order to optimize the selectivity for dibutyl oxalate.
The oxygen concentration in the reaction tower is kept below the explosion limit by gas circulation, which also improves the carbon monoxide utilization and gas–liquid contact rate.
The solution released from the tower is flashed to evaporate the carbon dioxide gas formed as a byproduct. The water formed during the reaction is removed by azeotropic distillation with butyl nitrite, and most of the solution is recycled to the reaction tower.
Part of the solution is further processed to yield pure dibutyl oxalate, which is obtained after the separation of catalysts, butyl nitrite, and butanol.
The palladium catalyst, butyl nitrite, and butanol are recovered and returned to the circulation liquid, which is also supplied with make-up butanol and nitric acid. The circulation liquid is then pressurized and fed back to the reaction tower.
The refined dibutyl oxalate is mixed with the mother liquor from the oxalic acid crystallization and hydrolyzed at about 80 °C into oxalic acid and butanol.
The oxalic acid solution and butanol are separated by phase separation. Oxalic acid dihydrate is then crystallized from the oxalic acid solution, and butanol is recycled to the process after refining.
2. Chemical Reactions of oxalic acid
The behavior of oxalic acid is unique due to the interaction between neighboring carboxylate groups, resulting in an increased dissociation constant and ease of decarboxylation.
Upon rapid heating to 100°C, oxalic acid decomposes into carbon monoxide, carbon dioxide, and water, with formic acid as an isolable intermediate.
Decomposition of oxalic acid in aqueous solutions can be induced by light and, to a greater extent, by γ- or X-rays, resulting in carbon monoxide, carbon dioxide, formic acid, and occasionally hydrogen. Heavy metal salts, such as uranyl salts, catalyze this decomposition.
Oxalic acid cannot form an intramolecular anhydride, but can be decomposed into carbon monoxide, carbon dioxide, and water upon heating to over 190°C or warming in concentrated sulfuric or phosphoric acid. This decomposition is not exothermic.
The reducing properties of oxalic acid form the basis for its practical applications, as it can easily be oxidized to the harmless end products carbon dioxide and water by a variety of oxidizing agents, including air and the salts of heavy metals.
Oxalic acid is easily esterified, producing acidic monoesters or neutral diesters as intermediates in chemical syntheses. These esters react readily with water, ammonia, or amines to produce the corresponding acyl derivatives.
The metal salts of oxalic acid also demonstrate important chemical characteristics, existing as either acidic or neutral salts. Alkali metal and iron (III) salts are readily soluble in water, while all other salts are sparingly soluble.
The nearly complete insolubility of alkaline-earth salts, especially calcium oxalate, finds applications in quantitative analysis. When heated, these metal salts lose carbon monoxide, and some easier decomposable salts also lose carbon dioxide. Alkali and alkaline-earth salts form carbonates under these conditions.
Metal salts of manganese, zinc, and tin form oxides, while iron, cadmium, mercury, and copper salts form mixtures of oxides and metals. Nickel, cobalt, and silver salts afford pure metals. Anhydrous fusion of oxalates with alkali yields carbonates and hydrogen.
3. Uses of oxalic acid
With regards to its applications as a synthetic intermediate, oxalic acid or its salts have also been utilized primarily as reducing agents, which produce harmless end products of carbon dioxide and water, as well as precipitants for calcium ions, or as complexing agents for the salts of heavy metals.
3.1 Metal Treatment
Heavy metals, particularly iron, form soluble double salts with sodium, potassium, or ammonium oxalates.
Iron (III) oxalate is the only readily soluble heavy metal salt. Oxalic acid salts are consequently employed for rust removal from cooling systems (such as automobile radiators), boilers, or steel plates prior to phosphating.
They can also be used to remove iron veins from marble, as a constituent of metal cleaners with rust protection, particularly for copper, silver, or aluminum, in anodizing of aluminum, and in the creation of very hard, abrasion- and corrosion-protective coatings, where the use of additives imparts different surface colors to aluminum.
Oxalic acid is also employed in cleaning materials for electronic devices and as coatings for protecting stainless steel, nickel alloys, chromium, alloy steels, and titanium.
3.2 Textile Treatment
Oxalic acid is utilized as a mordant in the printing and dyeing of wool and cotton. It is used as a stripping agent for wool colors to achieve special pattern effects, as an auxiliary in indigo caustic discharge printing, and in vat dyeing as a reducing agent for potassium dichromate.
In laundries, oxalic acid is used to remove rust stains and neutralize alkalinity, and it also has antibacterial properties.
Oxalic acid can be employed as a catalyst for cross-linking of textile finishing agents in the permanent pressing of cellulose fabrics and for flameproofing.
3.3 Bleaching Agents
Oxalic acid is utilized in the tanning and bleaching of leather, as well as in the bleaching of cork, wood (especially veneered wood), straw, cane, feathers, and natural and synthetic waxes.
The majority of oxalic acid is employed for bleaching pulpwood.
In leather processing, oxalic acid is used for whitening instead of sodium dithionite.
Reference
- Oxalic Acid; Ullmann’s Encyclopedia of Industrial Chemistry. – https://onlinelibrary.wiley.com/doi/10.1002/14356007.a18_247.pub2