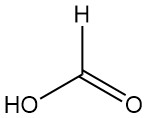
What is Formic Acid?
Methanoic acid, better known as formic acid, is the simplest carboxylic acid with the formula HCOOH. It is a colorless, corrosive liquid with a pungent odor that is completely miscible with water and many polar solvents but only partially miscible with hydrocarbons.
Formic acid derives its name from ants (latin. Formica), from which it was first obtained by dry distillation. The first scientific study on its properties, “Concerning Some Un-Common Observations and Experiments Made with an Acid Juice to be Found in Ants,” was published as early as 1670.
Table of Contents
1. Physical Properties of Formic Acid
Formic acid is a colorless, clear, corrosive liquid with a pungent odor. It is the strongest unsubstituted alkyl carboxylic acid.
The temperature dependence of the density of formic acid is given in Table 1.
Temperature, °C | Density, g/cm3 |
---|---|
0 | 1.244 |
10 | 1.232 |
15 | 1.226 |
20 | 1.220 |
25 | 1.214 |
30 | 1.207 |
40 | 1.195 |
50 | 1.182 |
60 | 1.169 |
70 | 1.156 |
80 | 1.143 |
90 | 1.130 |
100 | 1.117 |
Formic acid does not increase in volume when it solidifies and has a tendency to undergo supercooling.
Table 2 shows the vapor pressure curve of pure formic acid. The vapor of formic acid deviates considerably from the behavior of an ideal gas because the molecules dimerize partially in the vapor phase. At room temperature and normal pressure, 95% of the formic acid vapor consists of dimerized formic acid.
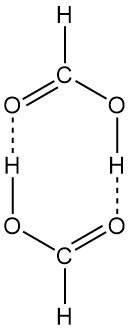
Liquid | Solid | ||
---|---|---|---|
Temperature, °C | Pressure (kPa) | Temperature, °C | Pressure (kPa) |
-5.23 | 1.083 | -5.07 | 0.664 |
0.00 | 1.488 | 0.00 | 1.096 |
8.25 | 2.392 | 8.25 | 2.392 |
12.57 | 3.029 | - | - |
20.00 | 4.473 | - | - |
29.96 | 7.248 | - | - |
39.89 | 11.357 | - | - |
49.93 | 17.347 | - | - |
59.98 | 25.693 | - | - |
70.04 | 37.413 | - | - |
79.93 | 52.747 | - | - |
100.68 | 101.667 | - | - |
110.62 | 135.680 | - | - |
The enthalpy of the gas-phase dimerization is -63.8 kJ/mol.
The ring-type dimeric structure exists both in the vapor phase and in solution. Liquid formic acid consists of long chains of molecules linked to each other by hydrogen bonds. Solid formic acid can also be isolated in two polymorphic forms (α and β).
Formic acid and water form a maximum-boiling azeotropic mixture whose boiling point is 107.6 °C at 101.3 kPa; it consists of 77.6 wt% formic acid and 22.4 wt% water.
The variation of dynamic viscosity with temperature is shown in Table 3. The dynamic viscosity of formic acid-water mixtures decreases approximately linearly as the water content of formic acid increases. The thermal conductivity of formic acid is markedly higher than that of comparable liquids because of its pronounced polarity.
Temperature, °C | Viscosity, mPa.s |
---|---|
10 | 2.262 |
20 | 1.804 |
30 | 1.465 |
40 | 1.224 |
50 | 1.025 |
The physical properties of formic acid are listed in the table below:
Property | Value |
---|---|
CAS number | [64-18-6] |
Formula | HCOOH |
Molecular weight | 46.03 g/mol |
Melting point | 8.3 °C |
Boiling point | 100.8 °C |
pKa | 3.74 |
Heat of fusion | 276 J/g |
Heat of vaporization (at boiling point) | 483 J/g |
Dielectric constant |
Liquid (at 20 °C): 57.9 Solid (at -10.1 °C): 11.7 |
Refractive index | 1.37140 |
Surface tension σ |
(at 20°C): 37.67×10-3 N/m (at 40 °C): 35.48×10-3 N/m (at 60 °C): 33.28×10-3 N/m (at 80 °C): 31.09×10-3 N/m |
Heat of formation |
Liquid (at 25 °C): -425.0 kJ/mol Vapor (at 25 °C, monomer): -378.57 kJ/mol (at 25 °C, dimer): -820.94 kJ/mol |
Heat of combustion | Liquid (at 25 °C): -254.8 kJ/mol |
Entropy |
Liquid (at 25 °C): 129.0 J K-1 mol-1 Vapor (at 25 °C, monomer): 248.88 J K-1 mol-1 (at 25 °C, dimer): 332.67 J K-1 mol-1 |
Heat of neutralization | 56.9 kJ/mol |
2. Reactions of Formic Acid
Even though formic acid is relatively stable at room temperature, it is actually a thermally unstable compound that has two major alternative routes for decomposition: dehydration and dehydrogenation reactions.
HCOOH → CO + H2O
HCOOH → CO2 + H2
The dehydration reaction, which is predominant in the liquid phase, is favored in the presence of oxide catalysts or strong acids, while the dehydrogenation reaction is promoted by metal catalysts.
Formic acid becomes particularly unstable in concentrations near 100%, and this must be taken into account while storing highly concentrated formic acid. In general, the decomposition of formic acid is a function of both acid concentration and temperature.
Formic acid exhibits many of the typical chemical properties of the aliphatic carboxylic acids, e.g., esterification and amidation, but, as is common for the first member of a homologous series, there are distinctive differences in the properties of formic acid and its higher homologues.
Formic acid forms esters with primary, secondary, and tertiary alcohols. The high acidity of formic acid makes the use of mineral acid catalysts unnecessary in simple esterifications. Primary and secondary alcohols are esterified in pure formic acid 15 000–20 000 times more rapidly than in pure acetic acid.
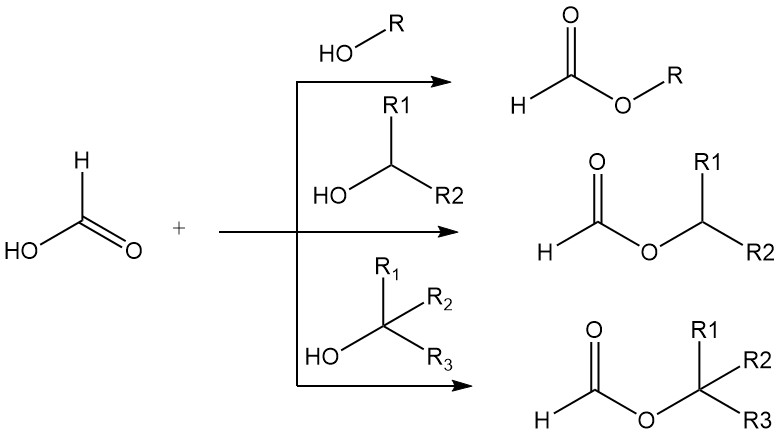
Formic acid also adds to the double bonds of olefins to form esters. Acetylenes react with formic acid in the vapor phase to yield vinyl formates.
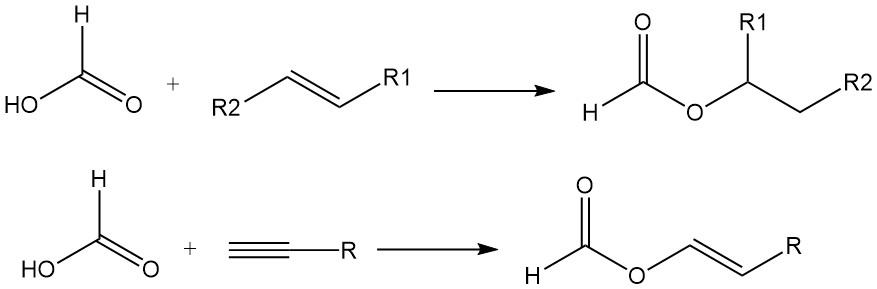
Formic acid reacts with most amines to form formylamino compounds. For example, in the production of formamides, formic acid is used to achieve formylation by dehydration. With certain diamines, imidazole formation occurs. Both reaction types have synthetic utility.
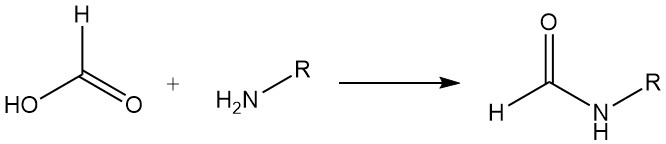
Formic acid and tertiary organic bases form addition compounds (3:1 and 2:1 ratios of formic acid to base). Addition compounds of formic acid and trimethylamine or triethylamine can be used as liquid reducing agents in many selective reductions. The reduction of sulfur dioxide to sulfur is approximately quantitative.
Formic acid dissolves iron and zinc and corrodes most types of steel. The reaction with iron yields Fe(II) formate and hydrogen gas. Among common metals and alloys, aluminum, copper, and nickel show some resistance.
2 HCOOH + Fe → Fe(OOCH)2 + H2
Formic acid is most corrosive in relatively dilute solutions in water. For mild steel, the highest corrosion rate is observed around 20% solution concentration, at which the conductivity of the solution is also the highest. Some corrosion inhibitors can provide very good protection.
Formic acid reacts with hydrogen peroxide in the presence of an acidic catalyst to form unstable performic acid (HCOOOH).

The formyl hydrogen atom exhibits some aldehydic character, and formic acid reduces some salts and oxides to metals. It can be used as a reducing agent (hydride donor) also in many organic reactions, replacing H2.
The reduction of imines (Schiff bases) by formic acid has been known for a long time. Primary amines can be prepared from ketones, ammonia, and formic acid (Leuckart reaction).

Formic acid can also be used as a source of carbon monoxide. Highly branched carboxylic acids can be prepared from olefins and formic acid in the presence of sulfuric or hydrofluoric acid (Koch carboxylic acid synthesis). The reaction proceeds via addition of CO.
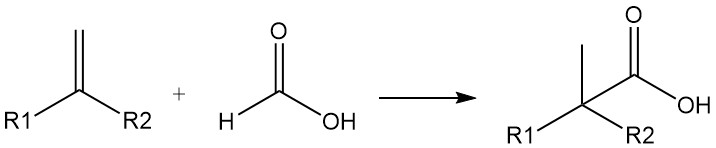
3. Production of Formic Acid
Formic acid is produced today mainly by two routes: the hydrolysis of methyl formate and the preparation of free formic acid from formate salts.
The methyl formate based process route is currently dominant. Approximately 90% of the installed capacity is based on this process. The economic disadvantages of the methods earlier practiced led to the development of a process specifically dedicated to the production of formic acid with no undesirable byproducts.
In the 1970s, the hydrolysis of methyl formate to methanol and formic acid was developed commercially by various companies into an economically feasible method.
This process involves carbonylation of methanol and subsequent hydrolysis of the methyl formate produced. The methanol resulting from this process is returned to the first stage.
Formic acid plants based on this process were started up at BASF (Germany) in 1981 and Kemira (Finland) in 1982. More recent large-scale producers using this route are the Chinese companies Feicheng Acid Chemicals and Luxi Chemical Group.
The other current production method involves the formation of free acid from its salts. Mainly sodium formate and calcium formate are used for this purpose. Acidolysis is normally carried out with sulfuric acid or phosphoric acid. Sulfate or phosphate salts are produced as byproducts.
3.1. Production of Formic Acid by Hydrolysis of Methyl Formate
The simplest theoretically possible method of producing formic acid is the reaction of carbon monoxide with water. Below 150 °C, the reaction is very slow, and although equilibrium is reached rapidly at higher temperatures, the pressure must be increased to obtain acceptable formic acid concentrations.
CO + H2O → HCOOH
The thermodynamic hurdles can be bypassed if methyl formate is formed as an intermediate.
The liquid-phase carbonylation of methanol to methyl formate in the presence of a basic catalyst such as sodium or potassium methoxide (NaOCH3 or KOCH3) and further hydrolysis to formic acid have been practiced industrially since the early 1980s.
Potassium methoxide is more soluble in methyl formate than sodium methoxide and, correspondingly, has a higher reaction rate. The methanol liberated in the second stage is returned to the first stage.
CH3OH + CO → HCOOCH3
HCOOCH3 + H2O → HCOOH + CH3OH
The methyl formate process is used, e.g., by Eastman Chemical Company, BASF, Kemira, Luxi Chemical Group, and Feicheng Acid Chemicals.
The reaction conditions for the first-stage carbonylation step are broadly similar in each, but they differ in their approach to the hydrolysis stage.
3.1.1. Carbonylation
According to the published data, typical reaction conditions appear to be 80 °C, 4.5 MPa pressure, and 2.5 wt% sodium methoxide catalyst. Under these conditions, the methanol conversion is around 30%, and the conversion of carbon monoxide is about 95%.
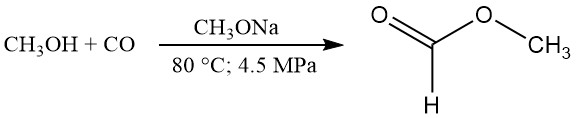
Nearly quantitative conversion of methanol to methyl formate can be achieved by recycling the unconverted methanol. The carbonylation rate can be increased by increasing the temperature, the CO partial pressure, the catalyst concentration, or the interface between the gas and liquid phases.
As a side reaction, the catalyst produces formate salts (Na and K). Formate salts are less soluble in methyl formate than in methanol. Hence, the risk of encrustation and blockage due to precipitation of catalyst must be managed.
The carbon monoxide must not contain a significant amount of carbon dioxide; otherwise, the catalytically inactive carbonate is precipitated.
The undecomposed alkali metal methoxide in the methyl formate must be neutralized to avoid the reverse reaction, that is, the decomposition of the methyl formate into methanol and CO when heated.
3.1.2. Hydrolysis
The hydrolysis equilibrium of methyl formate is relatively unfavorable but is dependent on the water concentration in a way that favors the use of a high stoichiometric excess of water, with consequent problems in finding an energy-efficient method of removing the excess water.
Formic acid acts as the hydrolysis catalyst (autocatalysis). One way to overcome the unfavorable position of the hydrolysis equilibrium is to hydrolyze methyl formate in the presence of an additive, e.g., a tertiary amine.
The base forms a salt-like adduct with formic acid; therefore, the concentration of free formic acid decreases and the hydrolysis equilibrium is shifted in the direction of the products. In a subsequent step, formic acid can be distilled from the base without decomposition.
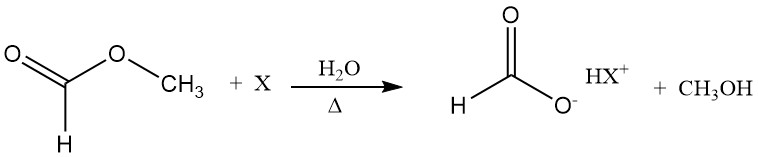
3.2. Production of Formic Acid from Formates
The reaction of formate salts with mineral acids (usually sulfuric acid) is the oldest commercial process for the production of formic acid, and it still has industrial importance. Perstorp is the largest producer of formic acid through the acidolysis of formate salts.
Sodium formate, potassium formate, and calcium formate are available industrially from the production of polyhydric alcohols.
The acidolysis of formate salts is technically straightforward, but the unavoidable production of coproduct salts such as sodium and calcium sulfate is a clear disadvantage of this route.
2 HCOONa + H2SO4 → 2 HCOOH + Na2SO4
(HCOO)2Ca + H2SO4 → 2 HCOOH + CaSO4
Some Chinese patents describe the preparation of formic acid from CO and alkali metal or alkaline earth metal hydroxides, followed by acidification with phosphoric acid. Carbon monoxide can come from phosphorus production. The resulting phosphate salts are used as fertilizers.
3.2.1. Formates as Polyol Byproducts
Formate salts occur as byproducts in the production of pentaerythritol, trimethylolpropane, and 2,2-dimethyl-1,3-propanediol (neopentyl glycol).
For example, pentaerythritol is produced by the reaction of formaldehyde with acetaldehyde in an aqueous alkaline medium. The intermediate aldehyde reacts further with formaldehyde by the Cannizzaro reaction to yield pentaerythritol and formic acid.
The formic acid formed reacts immediately with the alkali present, giving the corresponding formate salt as the final coproduct.
3 HCHO + CH3CHO → (HOCH2)3CCHO
(HOCH2)3CCHO + HCHO + H2O → C(CH2OH)4 + HCOOH
HCOOH + NaOH → HCOONa + H2O
2 HCOOH + Ca(OH)2 → (HCOO)2Ca + 2 H2O
Also, the excess alkali in the process is neutralized by formic acid. The pentaerythritol product is isolated by stepwise concentration and fractional crystallization.
3.2.2. Formates from Carbon Monoxide
Formate salts can be made by a direct process based on the reaction of CO with a base such as NaOH and KOH.
NaOH (aq) + CO → HCOONa (aq)
KOH (aq) + CO → HCOOK (aq)
3.3. Production of Formic Acid from Carbon Dioxide
Hydrogenation of carbon dioxide in alcohol produces formic acid in an almost water-free environment, which is an advantage in product recovery. Soluble Ru-complexes are the preferred catalysts, and a complexing agent is used to make the reaction thermodynamically favorable.
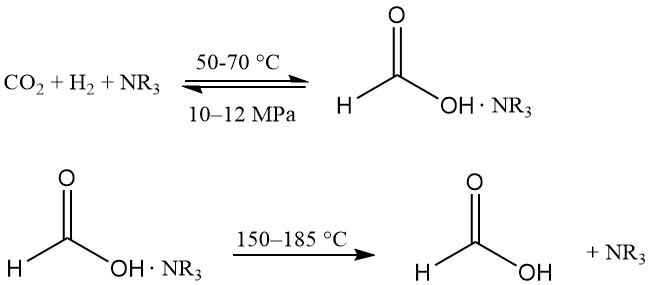
This technology was first introduced by BP Chemicals in the 1980s and has been developed by BASF.
The reaction takes place in a mixture of tertiary amine (usually n-trihexylamine) and alcohol (e.g., methanol) at 50–70 °C and 10–12 MPa. Some water is added to ease phase separation. The formic acid-amine complex is thermally dissociated at 150–185 °C.
The process must keep the expensive transition metal complex catalyst active but avoid even traces of it being present in active form in formic acid distillation because it can catalyze the decomposition of the acid. Possible catalyst residues can be reversibly inactivated with CO.
Several electrochemical processes for formic acid or formate salt production from carbon dioxide are in development. The process of CO2 electrolysis is basically running a fuel cell in reverse. In an electrolyzer CO2 is reduced at the cathode, while the oxygen evolution reaction takes place at the anode.
In recent years, great improvements in catalyst systems and electrodes have resulted in much higher yields, Faradic efficiencies, and fuel efficiencies.
3.4. Production of Formic Acid from Biomass
Virtually any plant biomass can be oxidized to formic acid, CO2, and water with high selectivity to formic acid by using polyoxometallates as oxygen-delivering catalysts.
Mineral acid hydrolysis of cellulose and hemicellulose around 200 °C produces a mixture of levulinic acid, formic acid, and furfural. The total reaction from C6 sugars to levulinic acid and formic acid is shown in Scheme 1.

3.5. Recovery of Formic Acid
3.5.1. Azeotropic Distillation
Formic acid-water mixtures cannot be concentrated to more than the azeotropic composition by simple distillation. The concentration of formic acid in the azeotropic mixture increases if distillation is carried out under pressure, but the higher boiling point at high pressure also increases the decomposition rate of formic acid.
A combination of distillation under pressure followed by vacuum distillation can produce practically all desired concentrations.
3.5.2. Extraction
Several technologies have been developed in which reactive extraction is used to transfer formic acid to a separate organic phase or formic acid is bound to a weak complexing agent in water phase. In both cases, the azeotrope can be avoided.
When phase separation is involved, it is possible to reduce the total amount of water that needs to be evaporated. Usually, secondary amides or tertiary amines are used.
In extractive distillation, formic acid is extracted in a distillation column by means of a basic extracting agent introduced counter-currently and fed into the bottom of the column. If this mixture is heated in a column down-stream, the formic acid is liberated from the salt-like adduct and distilled.
4. Uses of Formic Acid
Because of its acidity, aldehydic nature, and reducing properties, formic acid is used in a variety of fields. In contrast to mineral acids, formic acid evaporates without leaving any residue. In Europe, most of the formic acid is used in the feed industry and as a silage aid.
4.1. Uses of Formic Acid in Biomass Preservation
4.1.1. Silage
The term silage traditionally refers to the ensilation of forage crops (mainly grasses) for feeding bovines on farms. Consumption is dependent on climate; formic acid based ensiling is especially suitable for wet conditions. Northern Europe is the main consumption area.
Ensiling is based on fermentation under anaerobic conditions, whereby lactic acid produced by lactic acid bacteria preserves the silage. Lactic acid lowers the pH and thus prevents unwanted microbial growth.
The addition of formic acid results in a rapid initial drop in pH, which promotes the growth of lactic acid bacteria and suppresses the growth of bacteria that produce undesirable compounds such as butyric acid. When the pH drop is enhanced with formic acid, spontaneous fermentation is restricted.
Advantages include more residual sugars and protein. Restriction of fermentation is known to have a positive effect on voluntary intake in dairy cow feeding and thus enhance milk production.
Forage crops such as grass, corn, clover, and alfalfa are cut, chopped, and then fermented in silos or bales covered with airtight film. Formic acid is excellently suited to ensiling difficult-to-ensile materials, especially wet or low-sugar fodder plants, which may also have a high buffering capacity.
Formic acid is also used to restrict fermentation when ensiling crimped high-moisture grain.
Food and beverage industry byproducts, such as spent mash from breweries, can be preserved with formic acid solutions to provide long-shelf-life animal feed.
Formic acid is used in different formulations, sometimes in mixtures with other short-chain organic acids such as propionic acid, and is often buffered with a formate salt for handling safety and reduced corrosion.
4.1.2. Animal Biomass
Animal biomass can also be preserved with formic acid. The largest use is in processing fish-industry byproducts to produce fish silage. Proper treatment of fish offal is often required by environmental laws, and the production of fish silage can be an economically feasible solution.
Fish silage is a liquid, protein-rich product manufactured by lowering the pH of ground fish parts with an acidic preservative. Under these conditions, the enzymes from fish internals produce the final, long-shelf-life product in a few days.
Formic acid also enables efficient separation of fish oil during the ensiling process. The acid also acts as a processing aid by reducing microbial gas formation (“boiling”). Fish silage can be used as fish and pig feed or as a raw material for fish meal and oils.
Formic acid is the preferred acid in this application, usually combined with a suitable antioxidant. The application is a standard method in major salmon-producing countries, e.g., Norway, and is expected to gain more importance as aquaculture intensifies, especially in East and South-East Asia.
Slaughterhouse wastes can be preserved similarly to fish offal with acids, usually formic acid-lactic acid mixtures. The products are suitable for fur animals and, with limitations, for pet food.
4.2. Uses of Formic Acid in the Leather Industry
One of the biggest users of formic acid globally is the tanning industry. As the tanning industry has moved to lower-cost countries, the growth figures in Asia have been very high, compensating for the decline in Europe and North America.
China is the largest producer of leather, accounting for about 30% of world production.
Pretreatment of hides leaves them in a slightly alkaline state, but tanning requires acidic conditions. Therefore, the hides are treated with acid (typically sulfuric and formic acids) prior to tanning in a process called pickling.
Without this conditioning, the tanning agents would quickly become fixed at the surface of the hide, while its inner layer would remain raw.
Sulfuric acid reduces the pH of the liquor, while formic acid is capable of penetrating through the collagen fibers rapidly and homogeneously. It ensures that the tanning agent (usually basic chromium sulfate) will penetrate the entire thickness of the hide.
In leather dyeing, formic acid is used as a leveling agent to aid in moving the dye from one area of the leather to another, resulting in more uniform and smoother dye distribution.
4.3. Uses of Formic Acid in Textiles
In the textile industry, formic acid is used as a pH-regulating agent in dyeing wool, nylon, and other natural and synthetic fibers with acid and chrome dyes. In addition, formic acid is used to neutralize alkaline solutions and facilitate rinsing during laundering.
Improving living standards and increased fiber production, especially for export markets, are expected to increase demand for formic acid in textile dyeing and finishing in Asia.
4.4. Uses of Formic Acid as Feed Additives
Organic acids and salts have a long history in the feed industry, which commonly uses them as preservatives and for the acidification of piglet diets. Since 2006, when the EU banned antibiotic growth promoters (AGPs), the use of organic acids in feed has increased.
Formic acid has a strong acidification effect but also antimicrobial effects, which are used to protect feed and drinking water against bacterial contamination. Formic acid is very effective against Salmonella, Escherichia coli, and Campylobacter at pH 4.0.
It acts positively on the gut flora of animals and can improve both the apparent digestibility of energy and protein, the absorption and retention of some minerals. It seems to enhance the growth performance of weaned piglets and fattening pigs at lower dosages than other organic acids and salts.
In the poultry industry, formic acid has long been used to prevent the growth of pathogens in feed and feed materials. Blends of formic acid with propionic acid, lactic acid, or medium-chain fatty acids have broader antimicrobial effects than formic acid alone.
4.5. Uses of Formic Acid in Pharmaceuticals and Food Additives
Pharmaceuticals and food chemicals have been estimated to be the largest single sector of formic acid use in Asia (mainly in China).
Formic acid is used as a synthetic intermediate for various pharmaceuticals and food chemicals, including synthetic insulin (purification of recombinant insulin), caffeine, aspartame, and vitamin B1. It is also widely used for pH adjustment during the manufacturing of various chemicals.
Other applications in food include Salmonella decontamination and use as a preservative (E236, allowed in the USA but not in the EU, Australia, and New Zealand) and as a flavoring agent.
The use of formic acid in food preservation includes fumigation of fruit such as apples and cherries to reduce post-harvest decay. Formic acid is especially effective in destroying fungal spores on surfaces and containers in which fruits are stored.
In some food preservation applications, formic acid is blended with lactic and/or propionic acid. The mixture is minimally corrosive, but due to its low pH, it helps destroy harmful microorganisms and prevents their propagation, thus prolonging the shelf life of the product.
4.6. Other Uses
4.6.1. Rubber Coagulation
Formic acid is the preferred choice for coagulating latex, which is a suspension of microscopic natural rubber particles (polyisoprene) in an aqueous medium. The surfaces of the latex particles are charged, which creates repulsion between them, preventing coagulation.
In the coagulation process, formic acid neutralizes these charges, eliminating the repulsion. The process results in a consistent, high-quality natural rubber product. The use of stronger acids makes the pH drop too fast and inhomogeneously. As a result, the latex coagulates unevenly, which may affect its mechanical properties.
Weaker acids, such as acetic acid, are less efficient than formic acid and result in much higher acid consumption.
4.6.2. Gas Desulfurization
Formic acid is used as a desulfurization catalyst in flue gas desulfurization for coal-fired power plants. Sulfur, whose content in coal can be as high as 5%, is released as sulfur dioxide in the firing process.
Capturing sulfur dioxide by passing the flue gas through an aqueous limestone slurry results in gypsum (calcium sulfate). Adding formic acid to the desulfurization cycle increases the efficiency of sulfur separation.
4.6.3. Well Acidifiers
Formic acid is used in the stimulation of high-temperature wells in oil and gas fields when the conventional hydrochloric acid (HCl) systems cannot be adequately inhibited.
Well acidizing is achieved by pumping acid into the well to dissolve limestone, dolomite, and calcite cement between the sediment grains of the reservoir rocks.
Formic acid has the advantage of good inhibition against pipe corrosion at temperatures as high as 200 °C (possibly caused by a protective layer of decomposition products).
Mixed HCl-formic acid can offer further advantages. Formic acid does not dissociate in the presence of HCl, so there is no reaction with the carbonate until the HCl is virtually spent. HCl/formic mixtures can thus achieve greater penetration.
4.6.4. Formic Acid as Source of Hydrogen and Carbon Monoxide
Under hydrolysis conditions, the formate ion can form a bicarbonate ion and hydrogen.
HCO2– + H2O → HCO3– + H2
Both palladium and charcoal have been found to be active as catalysts.
The dehydrogenation reaction is promoted by metal catalysts.
HCOOH → CO2 + H2
There has been growing interest in formic acid- or potassium-formate-based hydrogen generation in fuel cells. Long operation times and instant recharge with no dependence on the electrical socket are the advantages when applied in portable devices.
The decomposition of formic acid in the presence of strong acids like sulfuric acid is a clean source of CO for carbon monoxide-based chemistry.
Formic acid or its salts can be used as a hydrogen source in chemical reactions without the formation of H2 as an intermediate (Leuckart reaction mechanism). The reaction, known as transfer hydrogenation, is selective and is catalyzed by many metals, even zinc powder.
When chiral catalyst complexes are used, formic acid can serve as a hydrogen source in asymmetric hydrogenation, a common reaction step in the synthesis of many fine chemicals and pharmaceuticals.
4.6.5. Cleaning Agents
Formic acid has some use as an active ingredient in commercial cleaning products such as descalers, rust removers, multipurpose cleaners and degreasers, and institutional laundry products. In descaling, calcium salt forms when calcium carbonate is dissolved by an acid.
The more readily soluble this salt is, the lower the risk of salt deposits that reduce acid effectiveness. In bathroom cleaners, it is claimed to combine the properties of an efficient descaling agent with those of a biodegradable biocide.
4.6.6. Solvent Use
Formic acid can be used to dissolve polyamides (e.g., nylon 66 and nylon 46) or silk to prepare fibers and membranes. It is also a useful component in semiconductor cleaning solutions.
5. Toxicology of Formic Acid
Formic acid exhibits a range of toxic effects depending on the route of exposure (inhalation, ingestion, or dermal contact) and concentration.
Acute Toxicity:
- Skin and Mucous Membranes: Formic acid is corrosive and can cause necrosis (tissue death) upon contact with skin and irritation/corrosion of mucous membranes at the point of entry (eyes, mouth, nasal passages).
- Ingestion: Methanol metabolism can generate toxic levels of formic acid, leading to metabolic acidosis. Oral ingestion of formic acid solutions can cause severe irritation and corrosion of the gastrointestinal tract.
- Inhalation: Inhalation of formic acid vapors irritates the respiratory system, with severity increasing with concentration. High concentrations can be fatal.
- Dermal Absorption: Formic acid can be readily absorbed through the skin, leading to systemic effects.
The LD50 after oral administration is reported to be 730 mg/kg in rats.
In rats, the LC50 is 7.85 mg/L after 4 hours of inhalation of the vapor. The predominant symptoms of inhalative intake are irritation of the eyes, irritation and corrosion of the nasal mucous membranes, and corneal opacity.
In rabbits, intravenous administration of buffered formic acid in the range of 0.46–1.25 g per kilogram of body weight leads to CNS depression; higher dosages cause convulsions. Subcutaneously administered doses of 0.8 g/kg in dogs and over 0.4 g/kg in cats lead to staggering, while a dosage of 0.4 g/kg leads to sleepiness in cats.
In dogs, lethal doses administered intravenously result in tonic and clonic convulsions.
Chronic exposure to formic acid at low concentrations in animal studies did not show significant effects on body weight or organ weight. However, it may decrease glutathione levels in the liver and kidneys.
Formic acid has not shown genotoxic potential in various in vitro and in vivo assays. However, some studies suggest a confounding effect of low pH at high concentrations, requiring further investigation.
Reference
- Formic Acid, Ullmann’s Encyclopedia of Industrial Chemistry. – https://onlinelibrary.wiley.com/doi/full/10.1002/14356007.a12_013.pub3