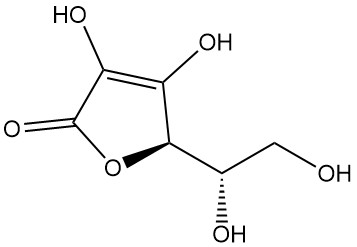
What is L-Ascorbic Acid?
L-ascorbic acid, also known as (2R)-2-[(1S)-1,2-dihydroxyethyl]-3,4-dihydroxy-2H-furan-5-one, is the IUPAC designation for vitamin C. It is a white or odorless powder or crystal with an acidic taste that is found in nature and has antiscorbutic activity, meaning it prevents scurvy, a disease caused by vitamin C deficiency.
L-ascorbic acid was first isolated in 1928, and its structure was determined five years later. Today, L-ascorbic acid is the most widely produced vitamin globally, with an estimated annual production of around 150,000 tons.
A key characteristic of L-ascorbic acid is its reversible oxidation to dehydro-L-ascorbic acid. This redox system allows L-ascorbic acid to act as a reducing agent.
Among the four diastereomers of ascorbic acid, only D-araboascorbic acid (also known as isoascorbic acid, erythorbic acid, and D-erythro-hex-2-enoic acid γ-lactone) shows weak antiscorbutic activity (about 5% that of L-ascorbic acid).
Table of Contents
1. Physical Properties of L-Ascorbic Acid
L-ascorbic acid is a white to very pale yellow crystalline powder that is highly soluble in water (1 g in 3 ml), moderately soluble in ethanol, propylene glycol, and glycerol, and insoluble in ether, chloroform, benzene, petroleum ether, oils, fats, and fat solvents.
A summary of the physical properties of l-ascorbic acid is given in the following table.
Property | Value |
---|---|
CAS number | [50-81-7] |
Formula | C6H8O6 |
Molecular weight | 176.13 g/mol |
Melting point | 190–192 °C (decomposition) |
Density | 1.65 g/cm3 |
Optical rotation | [α]20H2O: +20.5° to +21° (c = 1) |
[α]20EtOH: +52° (c = 0.5) | |
[α]20MeOH: +49.5° (c = 0.5) | |
pH | 3 (5 g/L) 2 (50 g/L) |
pK1 | 4.17 (3-OH) |
pK2 | 11.57 (2-OH) |
Redox potential | E = +0.127 V (pH=5) |
2. Chemical Properties of L-Ascorbic Acid
L-ascorbic acid exists in at least five tautomeric forms. The most prevalent form, tautomer (1), represents the crystalline structure and dominates in acidic aqueous solutions (pH = 2). Both the 2-OH and 3-OH groups are acidic, forming monobasic salts with bases; however, dibasic salts are unstable.
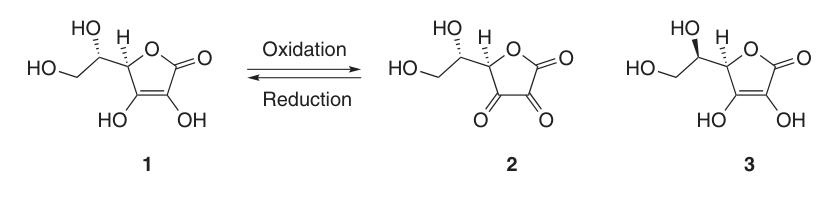
In sodium and calcium ascorbate, the metal ion interacts with the oxygen atom at position 3 (4a). The negative charge on the ascorbate monoanion is delocalized within the π-electron system, which contributes to the stability of its lactone ring in alkaline environments.
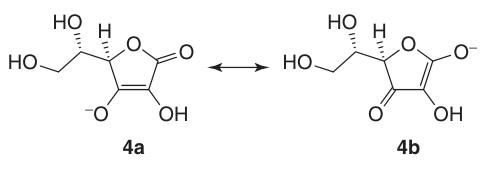
L-ascorbic acid readily undergoes isomerization to L-isoascorbic acid upon boiling in methanolic potassium hydroxide.
Due to its enediol structure, L-ascorbic acid acts as a potent reducing agent. The oxidation of ascorbic acid (1) yields dehydroascorbic acid (2), passing by the radical anion intermediate, semidehydroascorbic acid (5).
Semidehydroascorbic acid is a strong acid (pKa = 0.45) and a radical scavenger that can react with itself to regenerate ascorbic acid and compound (6) or react with other free radicals.
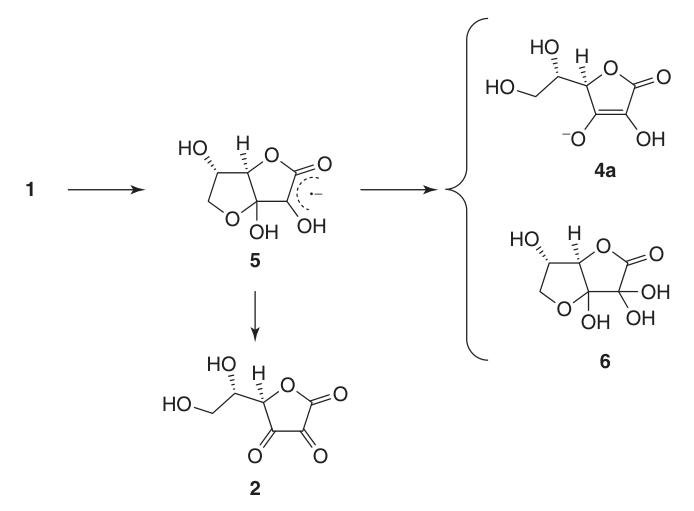
Transition metals like Cu and Fe catalyze the autoxidation of ascorbic acid to dehydroascorbic acid (2). These metals form a ternary intermediate complex with oxygen, which dissociates to yield dehydroascorbic acid (2), hydrogen peroxide, and the metal ion.
The autoxidation process is accelerated above pH 7 and can be inhibited by complexing metal ions with chelating agents like EDTA, metaphosphoric acid, citric acid, or oxalic acid.
Treatment of ascorbic acid with oxygen in methanol containing activated carbon forms a syrupy methanol adduct, which, upon further processing, provides dehydroascorbic acid (2) as a crystalline dimer in 64% yield.
At pH 2.5–5.5 in an aqueous solution and at 4 °C, compound (2) is stable for several days; however, reducing agents like hydrogen sulfide, dithiothreitol, or cysteine can quantitatively convert it back to ascorbic acid.
While dehydroascorbic acid (2) retains vitamin C activity, irreversible opening of the lactone ring to form 2,3-diketogulonic acid (8) leads to complete loss of this activity.
L-ascorbic acid undergoes degradation in aqueous solutions under both aerobic and anaerobic conditions. The rate of decomposition depends on factors like pH, temperature, light, concentration, and the presence of heavy metal catalysts.
The presence of oxygen significantly accelerates the degradation process compared to anaerobic conditions. The final oxidation products include L-threonic acid (9) and oxalic acid. Under anaerobic conditions, degradation leads to furfural (10) and CO2.
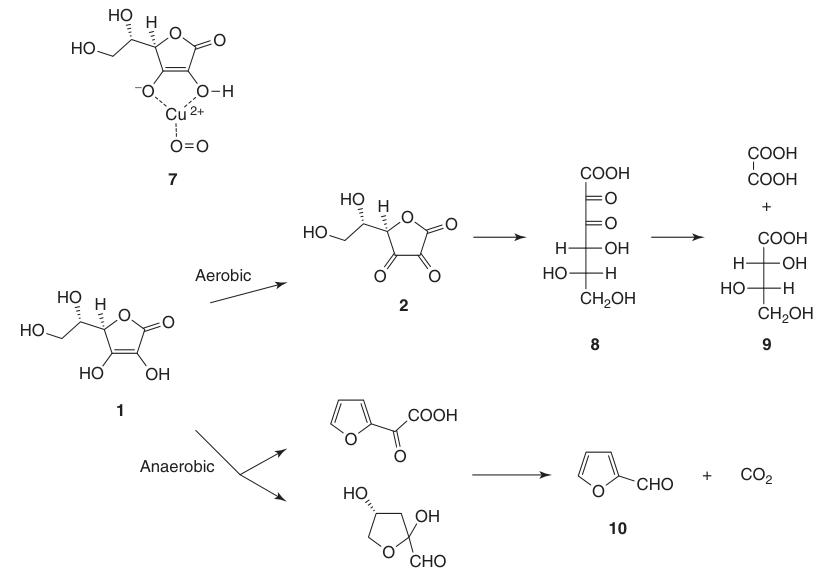
Solid L-ascorbic acid is very stable for a long time in the absence of moisture and light.
Protection against oxidative degradation in aqueous applications can be achieved by coating with fats and polymers or by chemical substitution of the enediol hydroxyl groups (L-ascorbate 2-sulfate, L-ascorbate 2-phosphate, and 2-O-methyl ascorbic acid).
3. Natural Sources of Vitamin C
Ascorbic acid (vitamin C) is a widely distributed compound found in plants and some animals. It is particularly abundant in green plants, with the highest concentrations observed in tropical fruits and gladiolus leaves. Its presence in yeast and non-cyanobacterial prokaryotes remains unclear.
Historically, during the winter months, vitamin C intake relied heavily on root vegetables like potatoes and cabbage. Today, with year-round access to fresh fruits and vegetables, dietary sources of vitamin C are more diverse. However, storage and cooking processes can contribute to vitamin C degradation.
The distribution of vitamin C within plant tissues varies. Notably, some plants, like Brassica species and Elaeocarpus spp., store vitamin C in bound forms, known as ascorbigen and elaeocarpusin, respectively.
Vitamin C is also found in animals, but at lower concentrations compared to plants. The highest levels are found in the endocrine organs of mammals like cows (hypophysis and adrenal glands).
Certain animal species, including humans, primates, guinea pigs, flying mammals, some birds, fish, and insects, lack the ability to synthesize vitamin C and rely on dietary intake.
Food Group | Vitamin C (mg/100 g) |
---|---|
Fish | 0–4 |
Asparagus | 7–25 |
Meat (beef, pork) | 0–10 |
Beans | 5–30 |
Milk (cow) | 1–2 |
Broccoli | 20–100 |
Fruits (apples, cherries, kiwifruit, oranges) | 4–180 |
Vegetables (broccoli, Brussels sprouts, cauliflower, spinach, turnips) | 20–90 |
Root vegetables (carrots, potatoes) | 2–20 |
Grains (oats, rye, wheat) | <1 |
Rice | 0 |
4. Industrial Production of L-Ascorbic Acid
The first successful synthesis of L-ascorbic acid occurred even before its complete chemical structure was determined. In this process, L-xylosone (11) was reacted with potassium cyanide to form l-xylonitrile (12), which, after lactonization and enolization, yielded L-ascorbic acid in around 40%.
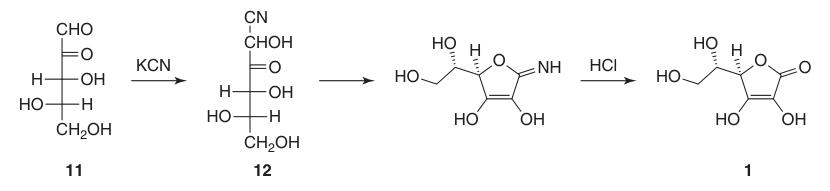
However, a major drawback of this initial synthesis was the difficulty and cost associated with obtaining L-xylosone (11).
Fortunately, about a year later, Reichstein and Grüssner published a more practical method for synthesizing L-ascorbic acid that uses readily available D-glucose (13) (a C6 sugar) as the starting material, and it became the dominant commercial production route for over 60 years, with minor refinements along the way.
Since the 1980s, the manufacturing process has undergone a significant shift. Sorbitol has become the preferred starting material, with a two-step fermentative oxidation being used to produce 2-ketogulonic acid.
While glucose hydrogenation remains a method for sorbitol production, it is not always performed by the final ascorbic acid manufacturer.
Despite the shift towards fermentation, the overall concept behind the modern process retains its connection to the original Reichstein synthesis. The key difference lies in the use of microbial oxidation instead of chemical methods.
4.1. Production of L-Ascorbic Acid by Reichstein Synthesis
The Reichstein synthesis of L-ascorbic acid is a series of simple, high-yielding chemical reactions that avoid carbon-carbon bond cleavage or formation.
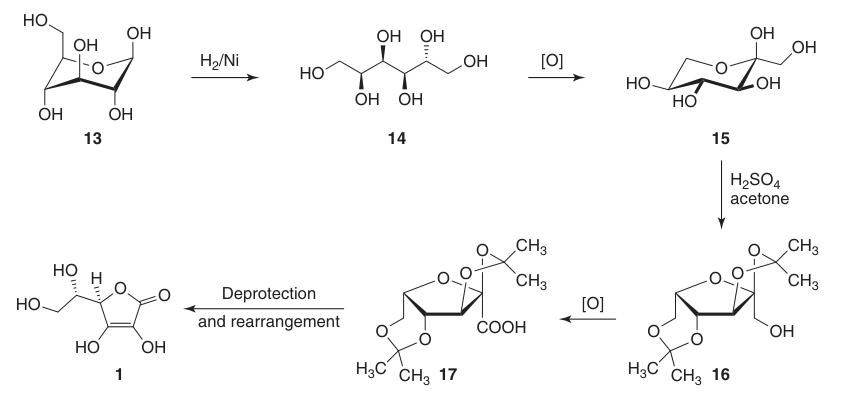
It reduces the C-1 position of D-glucose (13) and oxidizes positions 5 and 6, while maintaining the chirality at C-2 and C-3. This ultimately leads to the desired L-threo configuration at C-4 and C-5 in the final product.
The classical Reichstein-Grussner synthesis starts by reducing D-glucose (13) to D-sorbitol (14) using hydrogenation over a nickel catalyst. D-sorbitol is microbiologically oxidized to L-sorbose (15) by the bacterium Acetobacter xylinum.
L-sorbose (15) is treated with acetone and sulfuric acid at low temperatures to form the protected intermediate 2,3:4,6-di-O-isopropylidene-α-L-sorbofuranose (16).
This protection of hydroxyl groups at C-2, C-3, C-4, and C-6 allows for high-yield oxidation to di-O-isopropylidene-2-ketogulonic acid using potassium permanganate in an alkaline solution. The deprotection with hot water yields 2-keto-L-gulonic acid (18).
L-ascorbic acid is produced by heating 2-keto-L-gulonic acid (18) in water at 100 °C to give a 20% yield or by esterification followed by acidification to give a 70% yield.
The overall yield of L-ascorbic acid from D-glucose (13) using this method is around 15–18%.
4.2. Industrial Manufacture by the Reichstein Route
The transformation of Reichstein’s classic process into a commercially viable method involved significant optimization of each reaction step. These advancements encompassed both technical and chemical modifications and resulted in individual step yields exceeding 90%, leading to an overall yield of L-ascorbic acid from D-glucose (13) of approximately 60%.
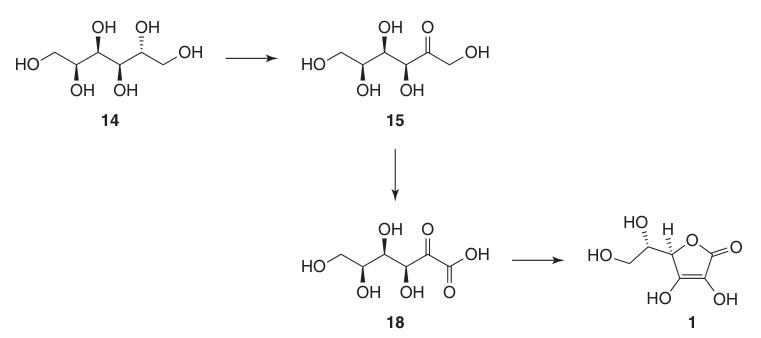
Key steps and optimizations are:
1. D-Sorbitol (14)
High-pressure hydrogenation of D-glucose (13) with a Raney nickel catalyst at elevated temperatures achieves near-quantitative yields of D-sorbitol (14) with minimal formation of byproducts like D-mannitol and L-iditol.
The sorbitol solution is directly used in fermentation without further purification, so high-quality starting glucose is needed.
2. L-Sorbose (15)
Microbial oxidation of sterile D-sorbitol (14) solutions using strains like Gluconobacter oxydans efficiently yields L-sorbose (15) with over 90% conversion.
Large-scale fermentations require strict control of pH (4-6), temperature (30–35 °C), and sterile conditions to minimize product loss during oxidation and workup by filtration and crystallization.
3. 2,3:4,6-Di-O-isopropylidene-α-L-sorbofuranose (16)
Protection of 2,3- and 4,6-hydroxyl groups in L-sorbose (15) is achieved by cyclic ketal formation with acetone and excess sulfuric acid as catalysts or dehydrating agents at low temperatures (e.g., 4 °C).
The main product is 2,3:4,6-di-O-isopropylidene-α-L-sorbofuranose (16), with monoisopropylidene derivatives as recoverable byproducts.
4. 2,3:4,6-Di-O-isopropylidene-2-keto-L-gulonic Acid (17)
Originally, oxidation of 2,3:4,6-di-O-isopropylidene-α-L-sorbofuranose (16) to 2,3:4,6-di-O-isopropylidene-2-keto-L-gulonic acid (17) used KMnO4 at elevated temperatures in dilute sodium hydroxide to yield around 90% product.
More cost-effective alternatives have been developed for continuous processes such as hypochlorite oxidation with catalytic nickel chloride/sulfate at 60 °C, which provides over 93% yield, and electrochemical oxidation using nickel/nickel oxide electrodes in alkaline solution.
Metal catalyst (palladium or platinum on carbon) oxidation with air or oxygen in an alkaline solution is another method that offers good yields.
5. L-Ascorbic Acid (1)
Conversion of 2,3:4,6-Di-O-isopropylidene-2-keto-L-gulonic acid (17) to L-ascorbic acid can be achieved through two methods:
- Deprotection to 2-keto-L-gulonic acid (18), followed by esterification with methanol and base-catalyzed cyclization.
- Direct acid-catalyzed cyclization of ascorbic acid from either the protected or deprotected 2-keto-L-gulonic acid (18).
The base-catalyzed method uses methyl 2-keto-L-gulonate (19), prepared from 2-keto-L-gulonic acid (18) by reaction with methanol in the presence of an acid. Subsequent reactions with bases like sodium hydrogen carbonate produce sodium ascorbate in high yield.
The first acid-catalyzed route to ascorbic acid emerged shortly after the discovery of the Reichstein process.
4.3. Modern Ascorbic Acid Production: Replacing Chemical Oxidation with Fermentation
The direct conversion of L-sorbose (15) to 2-keto-L-gulonic acid (18) (2-KGA), a crucial intermediate in vitamin C synthesis, has been a major target for improvement. While chemical oxidation using platinum or gold-platinum catalysts achieved some success, fermentation proved to be a more scalable and efficient approach.
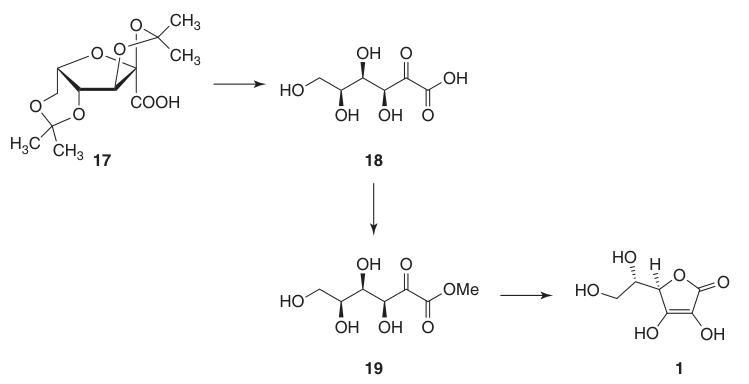
Early attempts explored using Gluconobacter for fermentative oxidation. However, a breakthrough came in the 1980s with the discovery of Ketogulonicigenium vulgare by the Chinese Academy of Sciences.
This microorganism offered the potential to completely replace the chemical oxidation steps in the Reichstein process. Today, virtually all global vitamin C production relies on 2-KGA (18) fermentation from L-sorbose (15) by Ketogulonicigenium vulgare.
The initial conversion steps from D-glucose to L-sorbose (15) remain largely unchanged from the Reichstein method developed in the 1930s. The subsequent conversion of L-sorbose to L-ascorbic acid now uses a two-step fermentation process that dominates worldwide:
- Commercially obtained D-sorbitol (14) is fermented with Gluconobacter oxydans to produce L-sorbose (15).
- The sterilized L-sorbose (15) broth undergoes further oxidation to 2-KGA (18) by a mixed fermentation involving Ketogulonicigenium vulgare and Bacillus megaterium. Bacillus megaterium plays a supportive role, enabling Ketogulonicigenium, which has limited nutrient requirements, to thrive on a simple and inexpensive medium.
This two-step fermentation process achieves rapid and efficient oxidation of L-sorbose (15) to 2-KGA (18), with reported titers exceeding 100 g/L and yields around 90% from L-sorbose within 40 hours.
Recent advancements have eliminated the need for intermediate L-sorbose sterilization in some processes.
Combining both fermentation steps into a single tank has been explored. Gluconobacter first oxidizes D-sorbitol (14) to L-sorbose (15), followed by Ketogulonicigenium growth and L-sorbose oxidation to 2-KGA (18).
This approach requires careful timing to prevent unwanted C-1 oxidation of D-sorbitol by Ketogulonicigenium’s enzyme. Despite promising results with 2-KGA (18) titers of 140 g/L and high conversion yields, the commercial viability of this single-tank process remains unclear.
The shift from chemical oxidation to fermentation has revolutionized vitamin C production, offering a more efficient, scalable, and potentially simpler process.
4.4. Other Methods for the Production of Ascorbic Acid
All production processes for l-ascorbic acid are partial syntheses in which the chirality at C-4 and C-5 is derived from naturally occurring sugars, primarily D-glucose. The conversion from D-glucose to L-ascorbic acid is achieved by manipulating the stereochemistry at C-4 and C-5 using carbon chain inversion or C-5 configuration inversion.
Historically, the Reichstein process dominated industrial L-ascorbic acid production. This multi-step chemical and fermentation approach has since been largely replaced by the more efficient 2-KGA (18) fermentation process.
While numerous alternative approaches have been explored, none have surpassed the economic importance of the 2-KGA (18) fermentation process. These alternatives include:
- Alternative 2-KGA (18) fermentation using microorganisms like Pseudomonas and engineered Gluconobacter strains.
- Producing L-ascorbic acid through oxidation and reduction steps on D-gluconic acid.
- Direct L-ascorbic acid fermentation using l-sorbosone dehydrogenase or engineered yeast/microalgae.
- Exploring cheaper options like pectin from fruit processing waste streams or starch.
- Chemical conversions to L-ascorbic acid intermediates, like starch to L-gulono-1,4-lactone, for potential combination with biocatalysis.
The focus for future advancements lies in fermentative and biocatalytic processes for simpler and more efficient L-ascorbic acid production. Promising areas include:
- Direct biocatalytic conversion with enzymes converting 2-KGA (18) or L-sorbosone directly to L-ascorbic acid.
- Genetically modified microorganisms such as engineer yeast or microalgae.
- Alternative feedstocks use cheaper and more abundant raw materials like starch or pectin waste streams.
Despite the dominance of the 2-KGA (18) fermentation process, continuous research strives to develop more sustainable, cost-effective, and streamlined methods for industrial L-ascorbic acid production.
4.5. Vitamin C from Natural Sources
While synthetic vitamin C dominates the market due to affordability and large-scale production, since 2010, natural vitamin C from acerola and rosa roxburghii fruits has become available, albeit at a higher price and lower production capacity.
These fruits naturally contain exceptionally high levels of vitamin C, about 2–3 g per 100 g of pulp.
Extraction processes yield products with a vitamin C content ranging from 10–25%, depending on the added excipients. Natural vitamin C is primarily marketed for human nutrition and personal care applications.
5. Industrial Applications of L-Ascorbic Acid
5.1. Uses of L-Ascorbic Acid in Pharmaceuticals and Food
Approximately one-third of total production is used for vitamin supplements in the pharmaceutical industry. The remaining portion primarily serves as a food and feed additive to enhance product quality and stability.
L-ascorbic acid is a common component in various pharmaceutical formulations, including multivitamins, tablets, syrups, elixirs, and effervescent tablets. Solid dosage forms are very stable, while liquid formulations are susceptible to degradation and interaction with other ingredients.
The food industry represents the largest application sector, particularly in meat, fruit, and flour processing. L-ascorbic acid functions as an antioxidant and essential vitamin, contributing to:
- Inhibition of nitrosamine formation in cured meats.
- Prevention of enzymatic browning in processed fruits and vegetables.
- Restoration and fortification of the nutritional value of food and beverages.
- Enhancement of flour and dough quality.
- Retardation of oxidative rancidity in fats and oils.
- Clarity improvement in wine and beer.
- Synergistic effects when combined with other antioxidants (e.g., tocopherols).
L-ascorbic acid, its salts, and ascorbyl palmitate or stearate are approved food additives in the European Community (E300 ff–304b) and classified as GRAS (Generally Recognized As Safe) by the FDA.
5.2. Uses of L-Ascorbic Acid in Animal Nutrition
While not essential for broilers, turkeys, and layers due to their endogenous production, vitamin C supplementation can positively impact poultry performance by improving weight gain, feed conversion, egg production, and eggshell strength.
L-ascorbic acid also contributes to enhanced health and immune competence, particularly under stressful conditions (e.g., heat stress), and it has synergistic effects when combined with vitamin E.
Vitamin C improves intestinal mucosa morphology and protects spermatozoa from oxidative damage in breeder males, leading to increased fertility.
Pigs can synthesize vitamin C, but efficiency is age-dependent. Piglets have limited production until 4-6 weeks, potentially benefiting from supplementation for performance and immune response.
Fish cannot synthesize vitamin C and require dietary intake. Ascorbic acid, or its more stable form, 2-phosphate ascorbic acid, is used in fish farming to prevent scurvy and bolster immune function.
Feed supplementation with vitamin C can be made with crystalline ascorbic acid, which can also be used in drinking water.
Special forms like ethyl cellulose-coated ascorbic acid or Na/Ca salts are used for heat- and moisture-treated feeds (pellets or extrusion).
Refer to Table 3 for detailed vitamin C requirements in various animals.
Animal | Recommended Supplementation |
---|---|
Laying hens | 100-200 |
Broilers for fattening (starter to finisher) | 100-200 |
Broiler breeders | 100-150 |
Turkeys for fattening (starter to finisher) | 100-200 |
Turkey breeders | 100-200 |
Piglets (prestarter and starter) | 100-200 |
Pig breeders | |
Gilts and sows | 200-300 |
Boars | 200-500 |
Calves | 200-500 |
Horses (mg/head/day) | |
Foals | 500-750 |
Race and breeding horses | 1000-2000 |
Salmon and trout | 150-250 |
Warm-water fish (carp and tilapia) | 150-250 |
Seabream and seabass | 150-250 |
Shrimp | 250-500 |
Dogs | 100-200 |
Cats | 100-200 |
5.3. Other Uses
Economic factors limit the application of ascorbic acid in plant science for seed germination, ozone protection, harvesting assistance, disease resistance, and growth regulation.
Minor applications exist in the polymer, photographic, and cosmetic industries.
Reference
- Vitamins, 14. Vitamin C (l-Ascorbic Acid); Ullmann’s Encyclopedia of Industrial Chemistry. – https://onlinelibrary.wiley.com/doi/10.1002/14356007.o27_o10.pub2