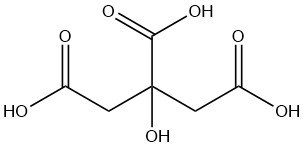
Citric acid is a weak organic acid found naturally in citrus fruits, especially lemons and limes. It is a colorless, crystalline solid at room temperature with the formula C6H8O7.
Citric acid, a tricarboxylic acid, was initially obtained and crystallized in 1784 by SCHEELE, through precipitation of calcium citrate with lime from lemon juice. The molecule’s three carboxyl and one hydroxyl groups were identified by LIEBIG in 1838.
In the United Kingdom, citric acid was first prepared from calcium citrate in 1860, and by 1880, its production from calcium citrate had begun inFrance, Germany, and the United States.
Citrus fruits are abundant sources of citric acid, with fruit containing between 5% to 9% of citric acid in the juice. Additionally, citric acid is present in various other fruits and is found in almost all plant and animal species.
The citric acid cycle, also called the Krebs cycle or tricarboxylic acid cycle, is crucial in the oxidation of sugars and acetates to carbon dioxide and water, releasing energy for physiological functions
Table of Contents
1. Production of Citric acid
Citric acid has been traditionally obtained from unripe citrus fruit for approximately 50 years.
In southern Italy, an entire industry was dedicated to this purpose with orchards and processing facilities. The procedure involved pressing the juice from unripe fruit and mixing it with lime (CaO) to precipitate calcium citrate.
The solid was then treated with sulfuric acid to precipitate calcium sulfate and form a solution from which citric acid was crystallized.
The yield of purified product was about 2-3 wt% of the fruit, and citrus oils were also obtained. However, this method has now been replaced by fermentation.
1.1. Production of Citric acid by Fermentation
Fermentation has been the most cost-effective method for producing citric acid since the 1930s. Strains of A. niger are employed in this process to convert carbohydrates to citric acid.
Both surface fermentation and submerged fermentation are used in large-scale production plants.
Some smaller plants use solid-state fermentation based on carbohydrates present in agricultural waste or byproducts.
The biochemical processes that lead to the excretion of citric acid have been the subject of studies by numerous researchers for over a century.
The theoretical yield of citric acid monohydrate from 100 kg of sucrose is 123 kg, or 112 kg of anhydrous acid. However, in the fermentation process, A. niger uses some sugar for growth and respiration, resulting in an actual industrial yield lower than the theoretical yield.
The yield varies between 60 and 85% of theoretical, depending on factors such as substrate purity, the specific strain of A. niger, and the control of fermentation.
1.1.1. Solid-State Fermentation
Solid-state fermentation for citric acid production involves spreading an agricultural residue containing carbohydrates in trenches and humidifying it with water.
Various agricultural residues, such as apple pomace, cassava bagasse, coffee husk, corncobs, grape pomace, wastes of kiwi, okara, orange, pineapple, and sugarcane, can be used as potential substrates for citric acid production.
Nutrients can be added to the water to support microbial growth. The trench is then inoculated with a suitable Aspergillus strain capable of converting the carbohydrates into citric acid.
Once the carbohydrates are depleted, the medium is washed with water to extract citric acid. The citric acid is then subjected to an appropriate recovery and purification process.
1.1.2. Surface Fermentation
Surface fermentation is a widely-used method for citric acid production, involving the growth of Aspergillus niger on a liquid substrate in vertically-stacked pans within a sterilized chamber.
The fermentation substrate, typically molasses or sugar syrup, contains a varying concentration of sugar, and inorganic nutrients are added, such as ammonium nitrate, potassium phosphate, magnesium sulfate, zinc sulfate, and potassium ferrocyanide.
The pH is adjusted between 3 and 7 depending on the carbohydrate source. Inoculation of the sterile substrate is performed with A. niger spores, which germinate and form a mat of mold on the liquid surface. After two to three days, the production of citric acid starts and continues at a constant rate until approximately 80-90% of the sugar has been consumed.
The fermentation proceeds slowly for an additional four to ten days before the pans are emptied and the mycelium is separated from the liquid. The mycelium is typically marketed as a feed ingredient, while the liquid undergoes a recovery process for further citric acid purification.
The chamber and pans are sterilized with steam, and moist and sterile air at a controlled temperature is introduced to cool the chamber during fermentation.
The substrate may be introduced manually or automatically, and may be supplemented with nutrients as required.
Sterilization can be batchwise or continuous, with the latter method consuming less energy and being faster.
1.1.3. Submerged Fermentation
Submerged fermentation is conducted in large fermentation vessels, which have become the preferred process for new investments since the latter half of the 20th century due to lower labor and smaller investment costs, as well as more compact construction areas.
The fermentation tanks are typically tall and narrow to maximize the oxygen transfer and may be equipped with mixing devices.
Mixing is especially important when hydrolyzing the carbohydrate feedstock in the fermentation vessel. For less viscous liquid feedstocks, airlift fermentation vessels are more economical.
Spargers, which are situated at the bottom of the vessel or under the stirrer, supply air that can be enriched with oxygen. The air passes through a sterile filter, and if necessary, it is cooled.
Because the process is exothermic, the vessel must be equipped with heat-exchange surfaces, which can be the outside walls or internal coils.
Ports are provided for introducing substrate, inoculum, and steam or other sterilizing agents; sampling and exhaust ports are also provided.
The substrate is prepared in a separate tank, and its pH is adjusted. The micronutrients may be added to this tank or directly to the fermentor. The substrate is sterilized by batchwise or, more commonly, continuous operation.
The fermentor is sterilized, charged with substrate, and inoculated. The actual fermentation process proceeds in two steps. A small part of the substrate is used to fill a seed fermenter, which typically contains one-tenth of the volume of a main fermenter.
After sterilization and cooling to 25–35 °C, spores of A. niger are added. The spores germinate, and mycelial pellets begin to develop in the first day of operation. In the meantime, a main fermenter is filled with substrate, sterilized, and cooled.
The content of the seed fermenter is then pumped into the main fermenter, where the conversion of the carbohydrates starts immediately. Fermentation in the main fermenter requires 3–7 days, depending on the substrate and process conditions used.
After fermentation is completed, the air supply is stopped to prevent the microorganisms from consuming the citric acid. The content is then pumped to rotary vacuum or belt press filters to separate the mycelium from the liquid.
As in the surface fermentation process, the mycelium is washed and is typically commercialized as a feed ingredient.
Nearly all citric acid worldwide is sold as crystalline products. To produce food-grade citric acid crystals, the citric acid is first isolated from the fermentation broth, followed by purification of the raw citric acid solution. Finally, the purified citric acid solution is crystallized and packaged.
2. Chemical reactions of citric acid
At a temperature of 175°C, citric acid undergoes dehydration to form aconitic acid, which can further lose carbon dioxide to yield itaconic anhydride. The latter can rearrange to citraconic anhydride or can add water to form itaconic acid.
Similarly, citraconic anhydride can be converted to citraconic acid upon addition of water. Mesaconic acid, the trans isomer of citraconic acid, can be obtained by evaporating a citraconic acid solution in the presence of nitric acid.
When citric acid is oxidized using potassium permanganate, 1,3-acetonedicarboxylic acid is obtained at 35°C while oxalic acid is formed at 85°C. Fusion of citric acid with potassium hydroxide yields a mixture of oxalic acid and acetic acid.
Citric acid can form mono-, di-, and tribasic salts with various cations, and the degree of hydration of these salts may vary. For instance, trisodium citrate can crystallize with either 2 or 5.5 molecules of water. The combination of different metal cations can give rise to complex salts, such as ZnNa3H(C6H5O7)2 and ZnNa4(C6H5O7)2.
Numerous metals can form stable complexes with citric acid, with some complexes, such as ferroammonium citrates, being able to crystallize.
The complex formed by copper and citric acid resembles the copper-tartaric acid complex of Fehling’s solution, and various classical methods have been employed to study these complexes.
Citric acid can chelate many metal ions in solution by forming bonds between the carboxyl or hydroxyl groups of the citric acid molecule and the metal ion.
Sometimes, more than one citric acid molecule is involved in the interaction with the metal ion. This property is useful for preventing precipitation, altering chemical potential, and modifying other chemical properties.
Citric acid can be esterified with many alcohols under normal conditions in the presence of a catalyst, such as sulfuric acid, p-toluenesulfonic acid, or an acid ion-exchange resin.
Catalyst-free esterification with alcohols boiling above 150°C is also feasible. For example, benzyl chloride and sodium citrate can produce di- or tribenzyl esters, and trimethyl, triethyl, and tributyl citrate are often employed as plasticizers in food-packaging materials.
Polyesters can be formed by reacting citric acid with dihydric alcohols, dihydric phenols, and polyhydric alcohols such as mannitol, sorbitol, and glycerol.
The esterification reaction can sometimes be halted before completion, leaving one of the carboxylic acid moieties free to form a salt. The resulting polyester may be soluble in water.
Citric acid can react with acid chlorides and anhydrides at the hydroxyl group, producing allyl esters of citric acid. Bis(citric acid esters) can be obtained from dicarboxylic acid chlorides.
Epoxides, including ethylene oxide, propylene oxide, and styrene oxide, can form polymers by reacting with citric acid or its esters at available hydroxyl and carboxyl groups.
Citric acid can react with ammonia, amines, amides, and carbamides in a manner similar to simple carboxylic acids. Aldehydes can also form adducts with citric acid, such as anhydromethylenecitric acid 5-oxo-1,3-dioxolane-4,4-diacetic acid.
3. Uses of Citric acid
Citric acid and its sodium or potassium salts are commonly used in various food products, including carbonated beverages, dry-packaged drinks, fruit drinks, jams, jellies, and canned fruits.
They also play a crucial role in preserving the color, flavor, and vitamin content of fresh and frozen vegetables and fruit, as well as in the production of vegetable oils.
In pharmaceutical applications, citric acid is predominantly utilized as an anticoagulant in blood storage and in effervescent tablets.
Citric acid is widely applied in cleaning processes, specifically in removing scale from boilers and heat exchangers due to its chelating ability.
Numerous industrial applications of citric acid benefit from its potent sequestering action with various transition metals, such as iron, copper, nickel, cobalt, chromium, and manganese.
Citric acid is commonly employed as a detergent builder, particularly in liquid formulations. Additionally, its solutions can eliminate sulfur dioxide from gases and chelate micronutrients in fertilizer.
Citric acid esters, such as trimethyl, triethyl, and tributyl citrate, are used as plasticizers for food-grade plastic containers.
Reference
- Citric Acid; Ullmann’s Encyclopedia of Industrial Chemistry. – https://onlinelibrary.wiley.com/doi/10.1002/14356007.a07_103.pub3