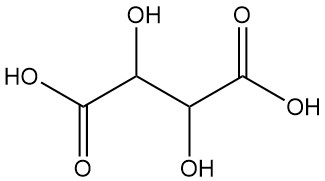
Tartaric acid, also known as acidum tartaricum, 2,3-dihydroxybutanedioic acid (IUPAC nomenclature), is a white crystalline organic acid found naturally in many fruits with the formula C4H6O6. The molecule possesses two asymmetric carbon centers with two carboxylic acid groups and a dialcohol in the same molecule, resulting in four stereoisomers.
Enantiomers:
- L(+)-Tartaric acid and D(-)-Tartaric acid are optically active isomers, rotating the plane of polarized light. These mirror-image molecules (enantiomers) share identical physical and chemical properties, except for opposite optical rotations.
Diastereomers:
- DL-Tartaric acid (racemic mixture) is a synthetic equimolar combination of L(+) and D(-) forms. It is optically inactive due to internal compensation.
- Meso-tartaric acid (unresolvable tartaric acid) is another optically inactive diastereomer with distinct physical properties compared to both the active and racemic forms. Neither racemic nor meso-tartaric are optically active acids.
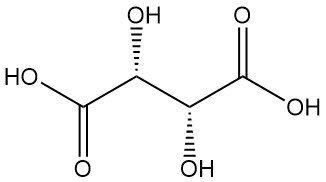
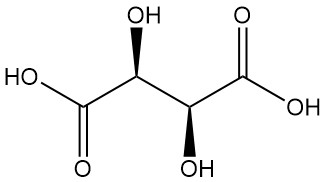
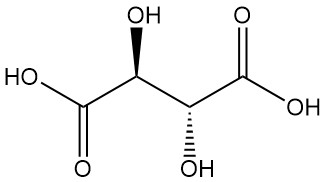
Tartaric acid, known since antiquity for its potassium salt (tartar), was first isolated by Scheele in the 18th century. Berzelius determined its structure in 1830. Pasteur’s groundbreaking work (1848–1860) on tartaric acid salts revolutionized organic chemistry.
He explained the connection between racemic and optically active forms by separating crystals of sodium ammonium tartrate and demonstrating their corresponding optical rotations.
L(+)-Tartaric acid is the predominant natural form and commercially produced variant. It is found as acid or salt in various plants, particularly grapes, where it accumulates as potassium bitartrate (tartar) during wine fermentation. This insoluble precipitate is the primary source for commercial L(+)-tartaric acid production.
Table of Contents
1. Physical Properties of Tartaric Acid
The physical properties of the natural L(+)-tartaric acid are given in Table 1, and they are identical to those of D(-)-tartaric acid.
Tartaric acid is an odorless solid with a strong acidic taste that is stable in the air.
Tartaric acid solutions have an optical rotation that varies depending on concentration, as represented by the following equation:
[α] =15.050-0.1535 c
where c is the concentration of tartaric acid in the range of 20–50% wt/vol.
Table 2 presents the solubility At various temperatures.
At various temperatures (°C), the relative density of tartaric acid solutions is listed in Table 3.
Property | Value |
---|---|
Molecular Mass | 150.09 g/mol |
Crystal Form | Anhydrous monoclinic needles (above 5 °C) |
Density | 1.7598 g/cm³ |
Melting Point | 169–170 °C |
Appearance | Colorless crystals |
Decomposition | Above 220 °C (caramelized sugar odor) |
Solubility | |
Water | Highly soluble |
Alcohol (18 °C) | 20.4 g per 100 g |
Diethyl Ether (18 °C) | 0.3 g per 100 g |
Optical Rotation at 20% wt/vol | +11.98° ( -11.98° for D(-)-tartaric acid) |
Enthalpy of combustion | 1149.9 kJ/mol |
Specific heat capacity (0-100 °C) | 1237 kJ kg⁻¹ K⁻¹ |
Acid dissociation constants (25 °C) |
K₁ = 1.17 × 10⁻³ K₂ = 5.0 × 10⁻⁵ |
Boiling points of solutions |
25% solution: 102.2 °C 50% solution: 106.7 °C |
Refractive index at melting point (170 °C) | 1.464 |
Temperature (°C) | 0 | 5 | 10 | 20 | 30 | 40 | 50 | 60 | 70 | 80 | 90 | 100 |
---|---|---|---|---|---|---|---|---|---|---|---|---|
Solubility (g/100 g H₂O) | 115 | 120 | 125 | 139 | 156 | 176 | 195 | 218 | 244 | 273 | 307 | 343 |
Temperature (°C) | Density |
---|---|
1 | 1.0045 |
10 | 1.0469 |
20 | 1.0969 |
30 | 1.1505 |
40 | 1.2078 |
50 | 1.2696 |
2. Chemical Reactions of Tartaric Acid
L(+)-tartaric acid melts between 170-180 °C and isomerizes (rearranges its structure) to metatartaric acid without water loss. Further heating yields amorphous anhydrides. These anhydrides revert to tartaric acid upon boiling with water.
Above 220 °C, tartaric acid decomposes, swells, and ignites, leaving a carbonaceous residue.
Tartaric acid is highly sensitive to oxidizing agents. Oxidation of tartaric acid by hydrogen peroxide and ferrous sulfate (Fenton reagent) produces dioxosuccinic acid.

Reduction with hydriodic acid yields succinic acid.
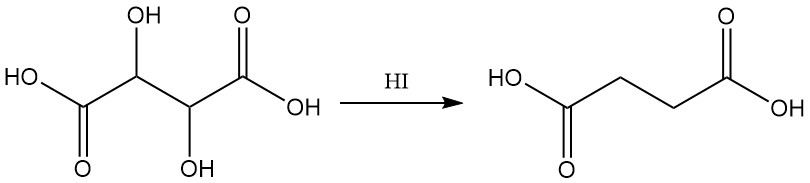
Tartrates in solution react with ammonio-silver nitrate to produce metallic silver.
Tartaric acid is used as a complexing agent to inhibit the precipitation of heavy metal salts by bases.
Prolonged boiling of L(+)-tartaric acid solutions with alkalis (KOH or NaOH) results in the formation of racemic and meso-tartaric acids.
3. Natural Resources of Tartaric Acid
L(+)-tartaric acid is the sole isomer commercially produced on a large scale. The exclusive source material comes from natural residues generated during winemaking. These residues primarily contain tartaric acid in the form of potassium bitartrate (cream of tartar) and, to a lesser extent, calcium tartrate.
Tartaric acid sources are as follows:
- Tartar is a reddish precipitate that deposits on the walls of wine vats as the ethanol concentration increases. It contains a high concentration (80–90%) of potassium bitartrate.
- Lees: These sediments settle at the bottom of wine vats and contain a lower concentration (19–38%) of potassium bitartrate.
- Dried Lees: These are processed lees with a higher (55-70%) potassium bitartrate content.
- Distillery Byproduct: Distilleries obtain this material from wine lees or grape marc (the leftover solids after grape pressing). After removing alcohol by distillation, the lees or marc are treated with calcium hydroxide to precipitate calcium tartrate.
4. Production of Tartaric Acid
While multiple tartaric acid isomers exist, L(+)-tartaric acid is the sole isomer produced commercially on a large scale. A very small amount of racemic acid is also synthesized for specific applications.
4.1. Production of L(+)-Tartaric Acid
The major producers of L(+)-tartaric acid are located in Spain, France, and Italy. For example, Société Legré-Mante, established in 1784, represents one of the oldest producers.
All methods for L(+)-tartaric acid production rely on the decomposition of calcium tartrate with sulfuric acid. Historically, two competing methods were employed to obtain the starting material (calcium tartrate): the Scheurer-Kestner acid process and the Scheele-Lowitz neutral process (including the Desfosses variant).
Currently, the more economical neutral process is the dominant method.
The dried starting material (tartar or lees) is first ground and then roasted at 160 °C for 2 hours in a roaster. This step removes organic impurities that could make the filtration difficult in later stages.
The hot roasted product is transferred to a reactor, where it is diluted with water and neutralized with calcium hydroxide to a pH of 5. The reaction temperature is maintained at 70 °C.
To improve reaction efficiency and minimize losses, an excess of 10% calcium chloride or calcium sulfate is added compared to the stoichiometric amount required. The following chemical reaction takes place:
2 KHC4H4O6 + Ca(OH)2 + CaCl2 → 2 CaC4H4O6 + 2 KCl + 2 H2O
The formed calcium tartrate is separated from the remaining liquid (mother liquor) by filtration using a rotary filter and then washed. If the starting material comes from distilleries, this initial stage may be bypassed.
In the second stage, the obtained calcium tartrate is decomposed in an aqueous solution using sulfuric acid. This reaction yields tartaric acid solution and insoluble calcium sulfate:
CaC4H4O6 + H2SO4 → H2C4H4O6 + CaSO4
An excess of approximately 5% sulfuric acid compared to the amount of tartaric acid is used to optimize the reaction. The resulting mixture is filtered and washed to remove impurities.
The red tartaric acid solution, with a concentration of around 200 g/L, is then concentrated in vacuum evaporators at 70 °C to reach a concentration of 650 g/L. Further concentration under vacuum at 70 °C occurs until crystals begin to form (at approximately 1300 g/L).
This liquid-crystal mixture is then transferred to crystallizers for slow cooling, promoting maximum crystal yield. After cooling, the mixture is dried in centrifuges to separate the crystals from the mother liquor.
The mother liquor, still containing tartaric acid, undergoes repeated cycles of evaporation, granulation, and drying to maximize the yield of granulated tartaric acid. The obtained granules are cooled to form a 650 g/L tartaric acid solution.
This solution undergoes further processing, including decolorization with activated carbon and chemical purification to remove excess iron and sulfuric acid, all performed at 70 °C. It is then filtered and concentrated under vacuum until crystallization starts.
The mixture is subsequently transferred to crystallizers or cooled to maximize crystal yield. The mixture is then separated by centrifugation. The mother liquor, containing residual tartaric acid, undergoes further cycles of evaporation, granulation, and drying before being retreated.
The purified granules are dried in an oven at 140 °C and then sieved into various grain sizes ranging from 2000 μm to less than 100 μm (powder).
While the process described above is the primary method, other production processes have been explored. These include the fermentation-based production of L(+)-tartaric acid and the chemical synthesis of racemic DL-tartaric acid.
4.2. L(+)-Tartaric Acid Production by Fermentation
While the conventional production method of L(+)-tartaric acid relies on chemical reactions, there’s ongoing research into fermentation-based alternatives.
Two main bacterial strains have been explored for this purpose:
- Acetobacter suboxydans: This bacterial species has been investigated for its ability to convert glucose into L(+)-tartaric acid.
- Nocardia tartaricans: This bacterium shows promise in converting cis-epoxysuccinic acid and its sodium derivatives into the desired product.
4.3. Chemical Synthesis of Racemic DL-Tartaric Acid

While L(+)-tartaric acid dominates the commercial market, a small-scale production process exists for racemic DL-tartaric acid.
This method starts with maleic acid or its salts. In an aqueous solution, maleic acid undergoes oxidation using 35% hydrogen peroxide in the presence of potassium tungstate as catalyst. This reaction yields epoxy-succinic acid, an intermediate product.
By boiling the reaction mixture, the epoxy-succinic acid hydrolyzes to form racemic tartaric acid. After cooling, the final product is isolated by centrifugation, washing, and drying.
Several variations of this process have been explored and documented in scientific publications. This research activity is further reflected in numerous patent applications filed for this method.
Currently, commercial production of racemic DL-tartaric acid remains limited, with only one small production unit operating in South Africa.
5. Derivatives of L(+)-Tartaric Acid
Tartaric acid readily forms numerous salts and esters, with some finding significant commercial applications. This section discusses the most well-known compounds.
5.1. Sodium Potassium Tartrate
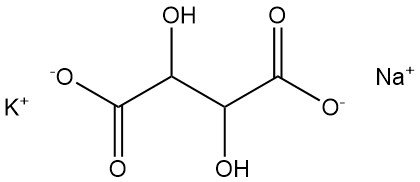
Sodium potassium tartrate, also known as Seignette’s salt or Rochelle salt, was discovered in 1672 by Pierre Seignette.
Physical Properties:
- Formula: KNaC4H4O6 · 4 H2O
- CAS Number: [6381-59-5]
- Molar Mass: 282.23 g/mol
- Density: 1.79 g/cm³
- Melting Point: 70–80 °C (decomposes at 220 °C)
- Solubility: 26 g/100 mL water at 0 °C, 66 g/100 mL water at 26 °C
The starting material for the production of sodium potassium tartrate is tartar with a minimum 68% tartaric acid content.
This solution is dilluted with water, neutralized with hot sodium hydroxide, decolorized with active charcoal, and then filtered. The filtrate is concentrated by evaporation, then crystallized by cooling to produce the solid salt, which is separated, washed, dried, and sieved before packaging.
Commercial grain sizes range from 2000 μm to < 250 μm (powder).
5.2. Potassium Bitartrate
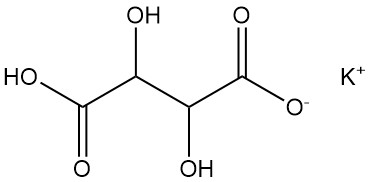
Potassium bitartrate is commonly known as cream of tartar.
Physical Properties:
- Formula: KHC4H4O6
- CAS Number: [868-14-4]
- Density: 1.96 g/cm³
- Solubility: 0.57 g/100 mL water at 20 °C, 6.1 g/100 mL water at 100 °C
It is primarily obtained from the mother liquor remaining after Rochelle salt production. Potassium bitartrate is decolorized, purified, filtered, acidified with hydrochloric acid or sulfuric acid, precipitated, centrifuged, dried, ground, and packaged as a fine powder.
5.3. Potassium Antimonyl Tartrate
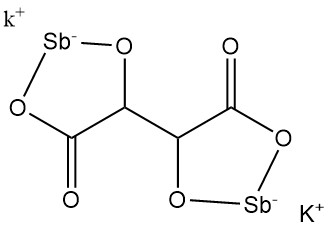
Potassium antimonyl tartrate (tartar emetic) was discovered in 1631 by Adrien de Mynsicht.
Physical Properties:
- Formula: KSbC4H2O6 · 1.5 H2O
- CAS Number: [28300-74-5]
- Molar Mass: 333.93 g/mol
- Density: 2.61 g/cm³
- Solubility: 8.7 g/100 mL water at 25 °C, 35.7 g/100 mL water at 100 °C
Potassium antimonyl tartrate is produced by the reaction of antimony oxide with the cream of tartar in water, followed by the concentration of the residual solution and crystallization. After filtration, the crystals are dried and pulverized.
5.4. Metatartaric Acid
Metatartaric acid is produced by heating tartaric acid to 170-180 °C. This conversion involves internal esterification within the tartaric acid molecules, leading to a more or less polymerized product. Metatartaric acid has exceptional inhibitory properties against the precipitation of tartaric acid salts.
6. Uses of Tartaric Acid
L(+)-Tartaric acid and its derivatives are used in various industries, including food, pharmaceuticals, viniculture, and others.
L(+)-Tartaric acid (E 334) is used:
- as an acidifying agent in wine musts, candies, jellies, jams, fruit nectars, ice creams, gelatins, and pastes, enhancing their flavor profiles.
- to preserve fruit, vegetables, or fish as a synergistic antioxidant, stabilizing pH, color, taste, and nutritional value.
- As antioxidant in greases and oils.
- In the preparation of carbonated beverages.
- As an excipient or carrier for active pharmaceutical ingredients, aiding in correcting basicity.
- As an acidifying agent for foaming tablets and powders due to its stability and high solubility
- As a retarder in the cement industry, particularly with plaster and gypsum.
- In polishing and cleaning metals.
Cream of tartar (E 336) is used to accelerate the precipitation of tartaric acid salts in wine, in the production of chemical yeasts and as a laxative in some drug formulations.
Seignette’s salt (E 337) is used in electroplating processes to improve deposition and yield, in electronics and piezoelectricity, as a reducing agent in the silvering of mirrors, and as a constituent of Fehling’s solution. It is also used as a combustion regulator in cigarette paper production, as a laxative in some pharmaceuticals, and as a mordant in the textile and leather industries.
In small quantities, potassium antimonyl tartrate is used as an expectorant in cough syrups and aids in treating some tropical diseases.
Metatartaric acid is used to inhibit tartrate crystallization in table wines.
Tartrates of fatty acid monoglycerides and diglycerides are used as emulsifiers in the bakery industry.
7. Toxicology of Tartaric Acid
Unlike citric acid, tartaric acid is not directly involved in the Krebs cycle (energy production pathway) in the human body.
L(+)-tartaric acid, the natural form, is found in fruits and wine. After oral intake, roughly 20% is eliminated through urine, while a significant portion undergoes bacterial degradation by the gut microbiota. No traces are detectable in feces.
Absorbed tartaric acid is rapidly eliminated from the bloodstream and either excreted by the kidneys or metabolized in various tissues.
Long-term studies in rats using L(+)-sodium tartrate haven’t revealed any signs of toxicity or carcinogenicity. Additionally, no negative effects on kidney function or pathology were observed at a daily dose of 3 g/kg body weight.
Racemic (DL)-tartaric acid has been less extensively studied, and research suggests it behaves differently from the natural L(+) isomer.
DL-tartaric acid is eliminated from the bloodstream at a slower rate and accumulates for a longer period in the kidneys, potentially causing weight gain in these organs. Due to this nephrotoxic effect, the DL-form is not approved for use in food or pharmaceuticals.
References
- Tartaric Acid; Ullmann’s Encyclopedia of Industrial Chemistry. – https://onlinelibrary.wiley.com/doi/10.1002/14356007.a26_163
- Tartaric Acid; Van Nostrand’s Encyclopedia of Chemistry. – https://onlinelibrary.wiley.com/doi/full/10.1002/0471740039.vec2453
- Organic Acids in Fruits. https://onlinelibrary.wiley.com/doi/abs/10.1002/9781119431077.ch8
- Tartaric acid. https://www.sciencedirect.com/science/article/abs/pii/B9780128190968000197