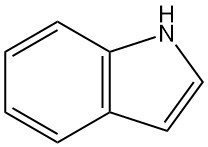
What is Indole?
Indole, also known as 1-benzo[b]pyrrole, is an aromatic heterocyclic organic compound that has a bicyclic structure (a benzene fused to a pyrrole) with the chemical formula C8H7N. It is a colorless solid with a very persistent musty odor.
Indole was first discovered by A. V. Baeyer and C. A. Knop in 1866 as a result of research on the reduction of isatin, which is derived from the natural dye indigo. Indole was synthesized by heating oxindole with zinc dust. In 1910, R. Weissgerber isolated indole from coal tar.
Indole has been found in Robinia pseudacacia, the jasmines, and certain citrus plants; in the perfume of Hevea bruziLiensis, in orange blossoms; and in the wood of Celtis reticulosa. It constitutes 2.5% of jasmine oil and 0.1% of orange blossom oil. When used in fragrances at higher concentrations, it exudes an intense fecal odor.
Indole is planar with 10 π-electrons in a completely conjugated system. The ring is classified as an π-excessive heteroaromatic compound because of the electron-donating character of the pyrrole-type nitrogen atom. The π-system is relatively electron-rich, particularly at carbon C-3.
Table of Contents
1. Physical Properties of Indole
Indole is a colorless, crystalline solid that is readily soluble in alcohol, benzene, ether, and most organic solvents but sparingly soluble in water. It is volatile with steam.
Indole is a neutral compound (nonbasic nitrogenous compound) but can be protonated or deprotonated by very strong acid or base, respectively. The pKa of the conjugate acid is about −2.4; that of the neutral compound is about 16.7.

Physical properties of indole are summarized in the following table:
Property | Value |
---|---|
CAS number | [120-72-9] |
Chemical formula | C8H7N |
Molecular weight | 117.15 g/mol |
Melting point | 52–54◦C |
Boiling point | 254 °C |
Density | 1.22 g/cm3 |
pKa | 16.7 |
Heat of combustion | 3.650 kJ/kg (at 25 °C) |
Enthalpy of vaporization (10.3–27.4 °C) | 597.5 kJ/kg |
Dipole moment | 2.11 D (benzene) |
2. Chemical Reactions of Indole
Indole as a heterocyclic compound shows reactivity characteristic of both pyrrole and benzene. The hydrogen atom attached to the nitrogen can be replaced by alkali metals. Oxidation of indole yields indigo, while mild hydrogenation produces 2,3-dihydroindole (indoline). Reactions with acids result in the formation of diindole, triindole, and polymer products.
2.1. Electrophilic Aromatic Substitution of Indole
Indole can undergo electrophilic aromatic substitution due to the electron-rich nature of the pyrrole moiety. The carbon in 3-position is the most reactive due to both electron density distribution and the stability of intermediate structures.
Computational studies using molecular orbital methods have revealed that the electron density at the 3-position is higher than at the 2-position. Moreover, the relative energies of the intermediate structures formed during electrophilic substitution suggest that the 3-position is more favorable for attack.
This preference is likely due to the retention of the benzenoid character of the carbocyclic ring in the intermediate formed at the 3-position.
In the case of 3-substituted indoles, electrophilic attack may still occur at the 3-position, even if it is already substituted. This can lead to a complex pattern of substitution, as either the new or the original substituent may migrate to the 2-position.
Many common electrophilic aromatic substitution reactions can be performed on indole. However, complications often arise due to the excessive reactivity of indole or the relative instability of the substitution products. One such example is halogenation.
2.1.1. Halogenation
Halogenation of indole can be achieved using various reagents, such as hypochlorite ion, sulfuryl chloride, pyridinium tribromide, and iodine. The primary product of halogenation is typically the 3-haloindole derivative.
Indole can be chlorinated using hypochlorite ion or sulfuryl chloride to yield 3-chloroindole. However, 3-chloroindole is relatively unstable in acidic aqueous solutions and can undergo hydrolysis to oxindole.

3-Bromoindole can be prepared using pyridinium tribromide as the source of electrophilic bromine. Indole reacts with iodine to give 3-iodoindole.
Both 3-bromoindole and 3-iodoindole are susceptible to hydrolysis in acidic conditions but are relatively stable in basic environments.
2.1.2. Nitration
Nitration of indole can be a complex reaction due to the formation of nitrogen oxides, which can participate in oxidative transformations. In strongly acidic media, nitration of 2-substituted indoles primarily occurs at the 5-position.

This is likely due to the formation of a conjugate acid intermediate, which has a reduced aromatic character and is more susceptible to nitration at the 5-position.
2.1.3. C-Alkylation
C-alkylation of indole can be achieved by reacting indole with alkylating agents under appropriate conditions. The regioselectivity of C-alkylation can be influenced by the reaction conditions and the nature of the alkylating agent.
Conditions that promote the formation of the electrophilic aromatic substitution intermediate, such as the use of strong bases or tight metal coordination at nitrogen or highly reactive alkylating agents like allylic and benzylic compounds, favor C-3 alkylation.
Unsaturated reagents activated by electron-withdrawing groups can also react with indole to undergo C-3 alkylation. An example is the reaction of indole with nitroethylene to form 3-(2-nitroethyl)indole.

Gramine, an intermediate formed by the reaction of indole with N,N-dimethylformaldiminium ion, can be used to introduce a variety of substituents at the 3-position. The dimethylamino group in gramine can be displaced by nucleophiles, or gramine can be converted to its quaternary salt before substitution.

2.1.4. Acylation
Acylation is another important method for introducing substituents onto the indole ring, particularly at the 3-position. Acylation can be achieved using various reagents, such as acyl halides and Vilsmeier-Haack reagents.
Highly reactive acyl halides, such as oxalyl chloride, can directly acylate indole without the need for a catalyst. Normal acid chlorides can be reacted with the magnesium or zinc salts of indole to produce acylation products.
The Vilsmeier-Haack reaction, involving an amide and phosphorus oxychloride, is a common method for acylation of indole.
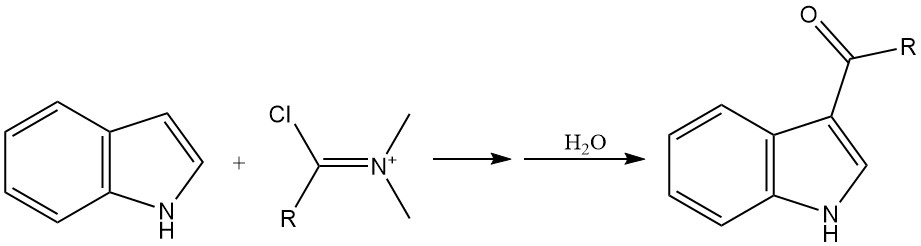
2.2. N-Alkylation of Indole
To facilitate N-alkylation, the indole ring must be deprotonated to generate the indole anion, which is a stronger nucleophile than the neutral indole molecule.
This deprotonation can be accomplished using various strong bases, such as sodium amide in liquid ammonia, sodium hydride, or potassium hydride in aprotic solvents (N,N-dimethylformamide and dimethyl sulfoxide), or phase-transfer catalysis.
The generated indole anion is alkylated by reaction with an alkyl halide or other alkylating agent. The choice of alkylating agent and reaction conditions can influence the regioselectivity of N-alkylation.

Primary, secondary, and tertiary alkyl halides can be used for N-alkylation of indole. However, primary alkyl halides are generally more reactive than secondary or tertiary alkyl halides. Other alkylating agents, such as dialkyl sulfates, alkyl tosylates, and alkyl triflates, can also be used.
2.3. Arylation of Indole
Arylation of indole is the introduction of an aryl group onto the indole ring. This reaction is typically accomplished through substituted intermediates rather than directly on indole. Palladium-catalyzed cross-coupling reactions have become the preferred method for arylation of indoles and other heteroaromatic rings.
Palladium-catalyzed cross-coupling reactions involve the coupling of an aryl halide or aryl triflate with indole in the presence of a palladium catalyst. The aryl nucleophile can be an indole-tin, indole-zinc, or indole-boronic acid.
Palladium-catalyzed cross-coupling reactions are highly selective, can be used to introduce a variety of aryl groups onto the indole ring, and are carried out under mild conditions.
2.4. Lithiation and Subsequent Transformations
Lithiation of indole is the introduction of a lithium atom onto the position 2 indole ring. Three intermediates have been frequently used, such as 1-phenylsulfonylindole (1), 1-t-butoxycarbonylindole (2), or lithium indole-1-carboxylate (3), and have achieved a good yield.
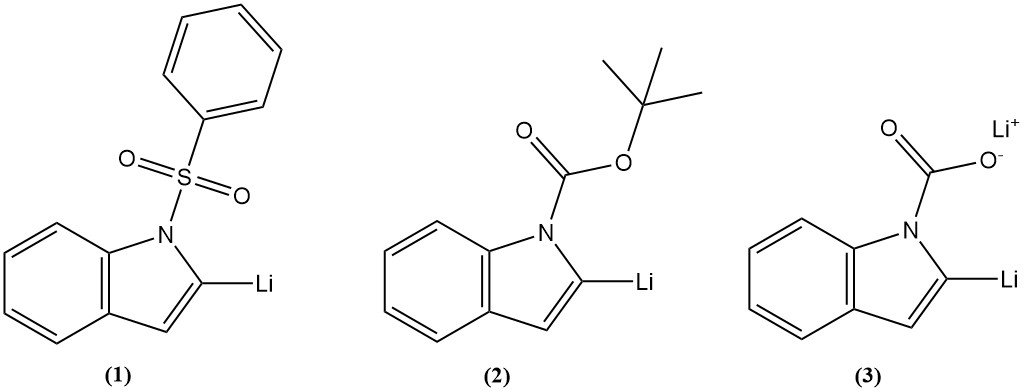
Lithiation of indole at the 2-position can be followed by reaction with various electrophiles to introduce a wide variety of substituents. Lithiation at other positions on the indole ring can be achieved by halogen-metal exchange.
2.5. Oxidation of Indole
Indole is susceptible to oxidation due to its electron-rich nature. Oxidation of indole can lead to the formation of various intermediates and products, depending on the oxidant used.
Oxidation of indole with oxygen often leads to the formation of 3-hydroperoxy-3H-indole intermediates. These intermediates can undergo further transformations, such as decomposition or rearrangement, to form various oxidation products.
Peroxycarboxylic acids can also oxidize indole to yield similar products as those obtained with oxygen. Other chemical oxidants, such as dimethyl sulfoxide in aqueous acid or MoO5·HMPA, produce oxindoles and 3-hydroxy-2-methoxyindolines, respectively.
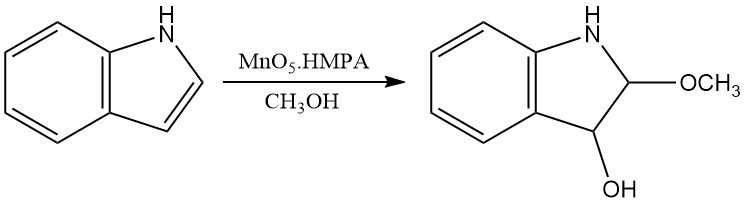
3. Production of Indole
Indole is primarily obtained from two sources: coal tar and chemical synthesis. Although synthetic methods have been described for the production of indole, extraction from the coal-tar distillate fraction is the only commercial source.
High-temperature coal tar contains approximately 0.2% indole. During coal tar distillation, indole is concentrated in a biphenyl-indole fraction that boils within a narrow temperature range of 245 to 255 °C.
After removing phenols and bases, indole is separated from biphenyl by:
- Reaction with potassium hydroxide to form its potassium salt, which can be separated from biphenyl.
- Azeotropic distillation with diethylene glycol.
- Extraction from the biphenyl-indole mixture using selective solvents like glycols, aqueous dimethyl sulfoxide, or monoethanolamine.
The crude indole obtained from these processes can be further purified by crystallization from aliphatic hydrocarbon solvents.
Several chemical methods have been developed for the synthesis of indole on a technical scale, including:
- Madelung synthesis involves the formylation of o-toluidine using formic acid, followed by cyclization to form indole.
- Dehydrogenating and cyclization of 2-ethylaniline to form indole.
- Cyclocondensation of aniline and ethylene glycol in the liquid or gas phase to produce indole.
- Cyclization of 2-(2-nitrophenyl)ethanol.
3.1. Production of Indole by Madelung Synthesis
The Madelung synthesis is a classic method for the preparation of indoles. This reaction is an intramolecular condensation of an o-alkylanilide. O-toluidine reacts with formic acid to produce N-formyl-o-toluidine, which is treated with sodium amide to form indole.

The Madelung synthesis has been used to prepare a variety of substituted indoles, including those with functional groups such as halogens, alkyl groups, and aryl groups.
The original Madelung synthesis used high temperatures and strong bases like sodium amide. For example, o-methylacetanilide can be converted to 2-methylindole by heating it with sodium amide at elevated temperatures.
In recent years, researchers have developed milder conditions for the Madelung synthesis, which can be advantageous for sensitive substrates or when avoiding side reactions is important.
One method involves using strong, non-nucleophilic bases like n-butyllithium or lithium diisopropylamide to form a dilithio derivative of the anilide, which can then undergo cyclization under milder conditions.
3.2. Other Synthesis Methods
Indole can be synthesized through various routes, including the reaction of aniline with ethylene bromide, heating o-amino-ω-chlorostyrene with sodium ethoxide, and heating the dianilide of tartaric acid with zinc chloride.
Indole-2-carboxylic acid, which yields indole by decaraboxylation, can be formed by heating o-formylphenylglycine with acetic anhydride and sodium acetate.
Other methods for indole synthesis include the distillation of oxal-o-toluic acid with zinc dust or its barium salt, pyrolysis of N-ethylaniline, catalytic dehydrogenation of o-ethylaniline, and heating of o,o’-diaminostilbene hydrochloride under reduced pressure.
Indole can also be obtained through the reduction of o-nitrophenylacetonitrile, o-nitrocinnamic acid, or o-nitrophenylacetaldehyde.
Finally, indole can be prepared by treating acetyl-o-aminocinnamic acid with hydrochloric acid at high temperatures or by reducing o-ω-dinitrostyrene. Heating indole-2-carboxylic acid and quinoline-2,3-dicarboxylic acid with calcium carbonate also gives indole.
3.3. The Fischer Indole Synthesis
The Fischer reaction has proved to be the most versatile for the synthesis of indole derivatives, although the reaction cannot produce indole itself. From a theoretical point, acetaldehyde phenylhydrazone would yield indole through the Fischer synthesis, but in reality none is obtained.
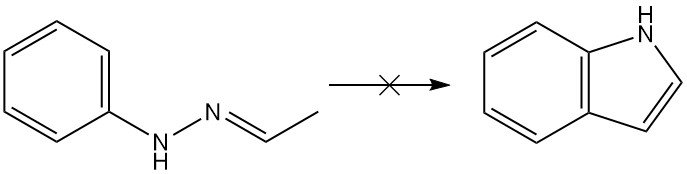
The Fischer indole synthesis is a classic method for the preparation of indoles that involves the cyclization of an N-arylhydrazone under acidic conditions to form an indole.
The key step in the Fischer indole synthesis is a [3,3] sigmatropic rearrangement of the enehydrazone tautomer of the hydrazone. For the cyclization to occur, there must be at least one α-hydrogen atom to the C-N bond. The regioselectivity of the reaction is influenced by the substituents on the hydrazone carbon.

If there is only one hydrogen atom α to the C-N bond, the product will be a 3,3-disubstituted-3H-indole. If both substituents at the hydrazone carbon have one or more α-hydrogens, product mixtures can result.
Generally, the more branched substituent is more likely to undergo cyclization. For example, phenylhydrazones derived from methyl alkyl ketones typically yield 2-methylindoles. However, the selectivity can be reversed under certain reaction conditions.
The presence of substituents on the phenyl ring of the hydrazone can also influence the regioselectivity of the Fischer indole synthesis. Meta-substituents can lead to the formation of isomeric indoles, while ortho-substituents make the reaction more complicated.
3.4. Biosynthesis of Indole from Tryptophan
One of the primary sources of indole in biological systems is the amino acid tryptophan. The conversion of tryptophan to indole occurs through a series of enzymatic reactions known as the tryptophan degradation pathway. This pathway is primarily found in bacteria and fungi, but it can also be active in certain mammalian tissues, such as the liver and intestine.
The initial step in this pathway is the cleavage of tryptophan by the enzyme tryptophanase. This reaction produces indole, pyruvate, and ammonia.
In addition to the tryptophanase pathway, tryptophan can also be degraded through the kynurenine pathway, which primarily is involved in the production of niacin (vitamin B3) but also produces indole derivatives.
4. Uses of Indole
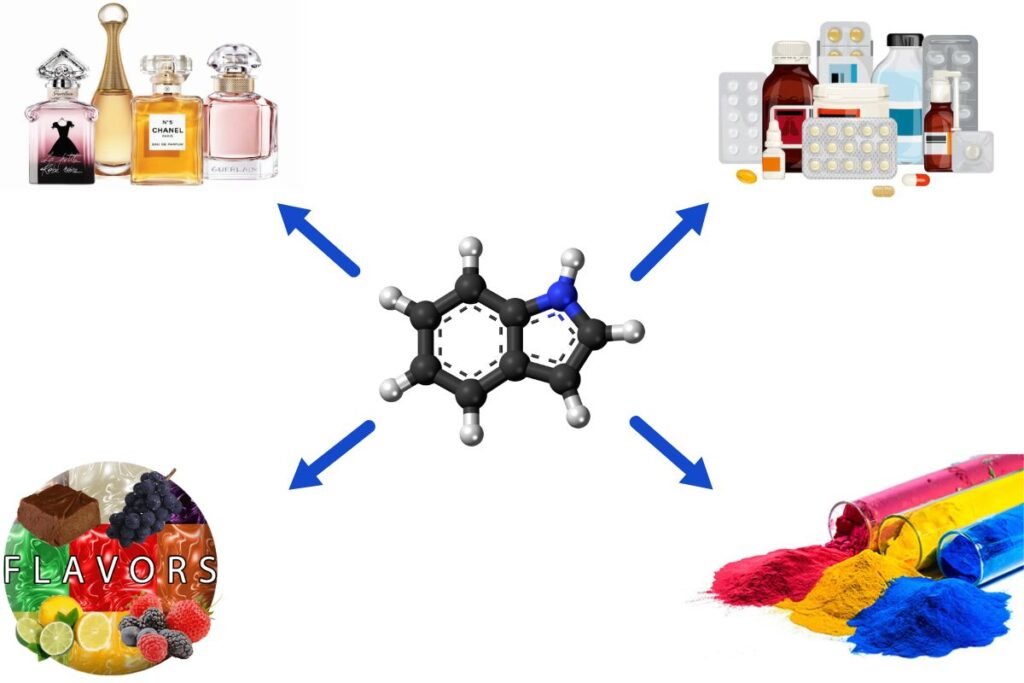
Indole is used in various industries, including pharmaceuticals, perfumery, and flavoring. It is also a natural component of several substances, such as tobacco smoke, jasmine oil, and orange-blossom oil. It is a bacterial degradation product of tryptophan in the gut.
Due to its pleasant aroma, indole has been used as a fragrance fixative for many years. It is also used as a synthetic flavoring agent.
Indole may act as a kairomone, a volatile chemical released by plants to attract phytophagous insects.
Indole is a precursor for the synthesis of the essential amino acid tryptophan. Both chemical and biotechnological methods can be used to produce tryptophan from indole.
Indole is used to synthesize plant growth regulators such as indole-3-acetic acid and indole-3-butanoic acid. Indoline, a derivative of indole, is used in the synthesis of fungicidal and bactericidal plant protectives.
Indole derivatives have a wide range of pharmaceutical applications, such as:
- Indomethacin and other indole derivatives are used as mild analgesics.
- Indoline and its derivatives are used in the synthesis of antihypertensive drugs.
- Tryptamine, a derivative of indole, is used in the synthesis of the vasodilator Vincamine.
- Pyridylalkylindoles, derived from indole, are used as antidepressants, antihistamines and antihypertensives.
- 3-peridinylindoles, obtained from indole, are used to prevent anoxia.
- 5-Chloroindole, a derivative of indole, is used in the production of tranquilizers, blood pressure-lowering drugs, and antiemetics, and in the treatment of Parkinson’s disease.
Indole and its derivatives are used as intermediates in the synthesis of various dyes, including cationic diazo dyes, cyanine dyes, indolylmethane dyes, and carbazole phthalein dyes.
5. Toxicology of Indole
The general population may be exposed to indole through inhalation of ambient air or tobacco smoke, ingestion of contaminated food, and dermal contact with vapors, food, perfumes, or other products containing indole. Occupational exposure can occur in workplaces where indole is produced or used.
Indole can be absorbed through various routes, including inhalation, ingestion, and dermal contact.
Indole absorbed from the gastrointestinal tract is metabolized in the liver to form indoxyl, which is then conjugated with sulfate to produce indican. These metabolites are excreted primarily through the urine.
Indole is readily absorbed from the gastrointestinal tract and undergoes rapid metabolism in the liver. The primary metabolites of indole include indican and oxindole, which are excreted primarily through the urine.
The acute oral LD50 of indole in rats and mice is approximately 1000–1100 mg/kg and 500 mg/kg, respectively. Autopsy studies following acute exposure revealed hemorrhages and hyperemia in internal organs and tissues. Indole has an unpleasant odor and can cause nausea at low concentrations.
Indole can cause slight temporary irritation to the skin and eyes when applied topically. Unlike its methylated derivative, skatole, indole does not induce pulmonary injury in cattle after repeated oral application.
High chronic doses of indole (20–200 mg/kg/day for more than three months) in mice, rats, rabbits, and dogs have been associated with hematological effects, including anemia, leukocytosis, and leukopenia. In cattle, hemolysis and hemoglobinuric nephrosis have been observed following repeated oral doses.
While there is no definitive experimental evidence for the carcinogenicity of indole, it may influence the formation of tumors caused by other agents. High doses of indole (800 mg/kg/day) have been shown to accelerate the development of skin tumors and bladder carcinomas in animal models.
Regarding genotoxicity, indole has been found to enhance the mutagenic effects of other agents in the Ames test. However, indole itself was not mutagenic in the Ames test or in a cell transformation test. In a DNA repair assay, indole caused reparable DNA damage in Bacillus subtilis.
Indole has been granted GRAS (generally recognized as safe) status by the Flavor and Extract Manufacturer’s Association (FEMA) and is approved for use as a flavoring material in food. However, there are no specific occupational exposure limits for indole established by regulatory agencies.
References
- Indole; Ullmann’s Encyclopedia of Industrial Chemistry. – https://onlinelibrary.wiley.com/doi/10.1002/14356007.a14_167
- Indole; Kirk‐Othmer Encyclopedia of Chemical Technology. – https://onlinelibrary.wiley.com/doi/abs/10.1002/0471238961.0914041519211404.a01
- Indole; Encyclopedia of Toxicology (Third Edition). – https://www.sciencedirect.com/science/article/abs/pii/B9780123864543008605
- Indole; Chemistry of Heterocyclic Compounds, Volume 8. – https://onlinelibrary.wiley.com/doi/10.1002/9780470186572.ch1
- https://www.sciencedirect.com/science/article/abs/pii/S092809871630207X
- https://www.sciencedirect.com/science/article/abs/pii/S0040403921007413