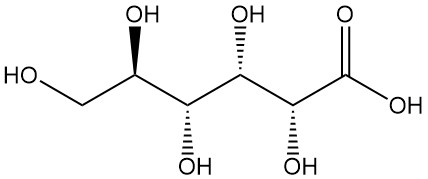
What is Gluconic Acid?
Gluconic acid, also known as 1,2,3,4,5-pentahydroxy pentane-1-carboxylic acid, is an organic acid with the formula C6H12O7. It is a white, odorless, crystalline powder that is naturally found in humans and other organisms and also in food products such as wine and honey.
Gluconic acid was first discovered in the 1870s by Hlasiwetz and Habermann during the oxidation of glucose with chlorine, and it was isolated in the form of its barium and calcium salts.
Later, researchers found that gluconic acid could be produced by treating various mono-, di-, and polysaccharides with oxidizing agents such as elemental halogen, copper (II) or hexacyanoferrate (III) salts, or mercury (II) oxide.
The byproducts of these reactions depend on the type of sugar and the oxidant used, but can include formic acid, glycolic acid, oxalic acid, and carbon dioxide.
In the 1880s, Butroux discovered that gluconic acid was produced, along with acetic acid, by the oxidative action of Acetobacter aceti on glucose, which was also found to be characteristic of other bacteria.
Molliard was the first to report the presence of gluconic acid in cultures of Aspergillus niger, which was formerly known as Sterigmatocystis nigra. The preferred method for producing gluconic acid and its derivatives today relies on Aspergillus strains, based on the work of several researchers.
Anodic oxidation was proposed in the 1930s as a method for preparing calcium gluconate, followed later by studies on the catalytic oxidation of glucose using air or oxygen.
Table of Contents
1. Physical Properties of Gluconic Acid
The crystallization of D-gluconic acid in its free form is difficult and According to some studies, the crystallization of the anhydrous substance is only possible below 30 °C, and that of the monohydrate at 0–3 °C.
The molecular mass of gluconic acid is 196.16 g/mol.
The anhydrous form of gluconic acid is a white, odorless, crystalline powder with a melting point ranging from 120 to 131 °C due to the formation of intramolecular anhydrides and a specific rotation of [α]D20 = -6.7° and [α]D25 = -5.4°.
It is highly soluble in water, slightly soluble in ethanol, and insoluble in nonpolar solvents. The commercial 50% gluconic acid aqueous solution has a pH of 1.82 and a density of 1.23 g/cm3.
D-gluconic acid forms lactones upon storage over desiccant at room temperature or heating above 50 °C and undergoes pyrolysis above 200 °C.
It exists in equilibrium with two lactones (1,5-lactone (1) and 1,4-lactone (2)) in aqueous solution and in the solid state. The free acid has a dissociation constant (KA) of 1.99 x 10-4 and a pKa of 3.70.
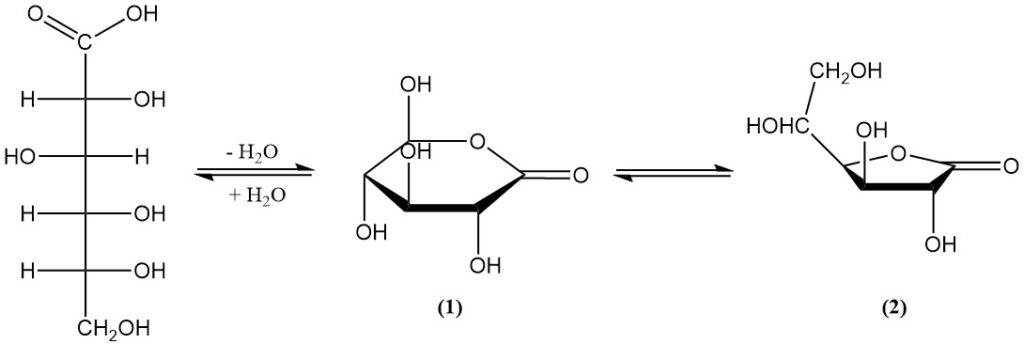
1,5-lactone (1) is a white, crystalline solid with a faint sweetness, a melting point of 153 °C, and a specific rotation: [α]D20 = +66.2°. It is highly soluble in water (and it increases with temperature) and slightly soluble in some organic solvents.
1,4-lactone (2) appears as fine needles with a melting point of 134–136 °C and a specific rotation: [α]D20 = +67.8°. It has low solubility in most solvents.
2. Chemical Reactions of Gluconic Acid
Oxidation of gluconic acid lactones or calcium salt with mild oxidants like nitric acid or hydrogen peroxide leads to a mixture of oxogluconic acids, primarily with ketone groups at positions 2 and 5.
Anodic oxidation, oxidation with sodium chlorate in an acidic solution, or fermentation by specific bacteria, like Acetobacter species, can produce 2-oxo-D-gluconic acid as the main product.

The reaction with concentrated nitric acid, or N2O4 converts gluconic acid to glucaric acid.

Hydrogenation of gluconic acid in water with a platinum oxide catalyst yields D-glucose in moderate yield, while 1,5-lactone gives a high yield.

Refluxing gluconic acid with concentrated hydriodic acid and red phosphorus produces hexanoic acid.

Functional groups of gluconic acid can theoretically react with various reagents (alcohols, acids, etc.); however, a complete reaction is necessary for stable derivatives. Partial reactions create unstable mixtures susceptible to hydrolysis and have limited practical value.
Gluconic acid and its alkali salts are valuable due to their ability to form stable complexes with polyvalent cations. Examples include gluconate salts of calcium, copper, iron, and manganese.
Nuclear magnetic resonance studies suggest that both carboxyl and hydroxyl groups are involved in complex formation with these cations.
3. Production of Gluconic Acid
D-gluconic acid is produced industrially by the oxidation of glucose or raw materials rich in glucose. The oxidation process can be chemical, electrolytic, catalytic, or biochemical.
3.1. Production of Gluconic Acid by Chemical Oxidation of Glucose
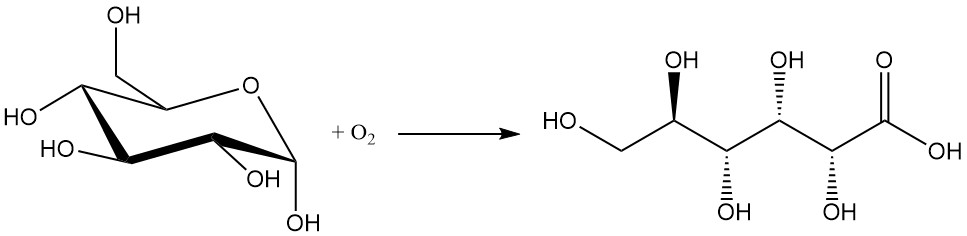
The chemical oxidation of glucose is the least preferred method due to its low yields (between 60 and 80%) and the formation of unwanted byproducts. The isolation and purification of the desired product are difficult.
Common oxidants used in this process include hydrogen peroxide, ozone, and oxygen.
3.2. Production of Gluconic Acid by Electrochemical Oxidation of Glucose
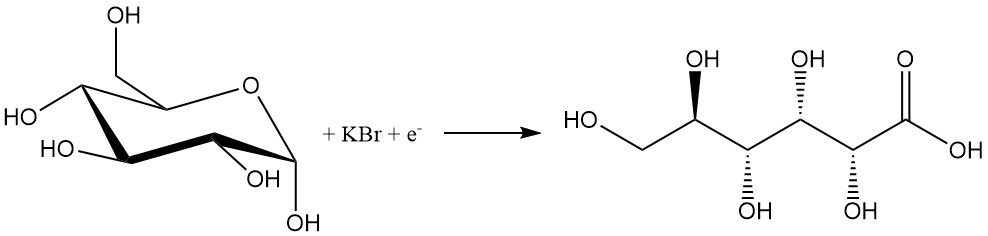
Similar to chemical methods, the electrochemical process has low selectivity due to the use of halogens.
A solution of glucose containing 10% bromide is electrolyzed at a current density of 1–20 A/dm2 to produce 80–97% gluconic acid. Bases like calcium carbonate or hydroxides are added to neutralize the resulting
acid.
The high cost of electricity makes this method less competitive.
3.3. Production of Gluconic Acid by Catalytic Oxidation of Glucose
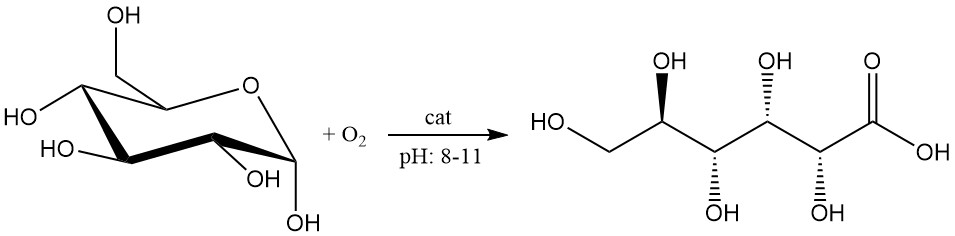
The catalytic oxidation of glucose has seen recent advancements and is considered promising for industrial scale production.
The glucose solution at a concentration of 1 – 2 mol/L is oxidized by oxygen or air at pH between 8 and 11.
Originally, platinum-group metals on carriers, such as activated charcoal and aluminum oxide, were used as catalysts. However, some challenges that have emerged using this type of catalyst include catalyst deactivation, the need for pure glucose, and byproduct formation.
Doping these catalysts with elements like lead, selenium, or bismuth improves activity and selectivity, and activated charcoal is the preferred carrier for these catalysts.
Using these catalysts to oxidize a 2 M glucose solution to gluconic acid with oxygen at 50 °C and pH 9.5, high yields (>99.5%) and purity (>99.5%) have been achieved with minimal purification needs.
These catalysts are also reusable, with minimal activity loss.
The economic viability of this method depends heavily on the cost, activity, selectivity, and lifetime of the catalyst, along with product purification and energy requirements.
Despite earlier suggestions, photochemical oxidation of glucose to gluconic acid is not viable.
3.4. Production of Gluconic Acid by Fermentation
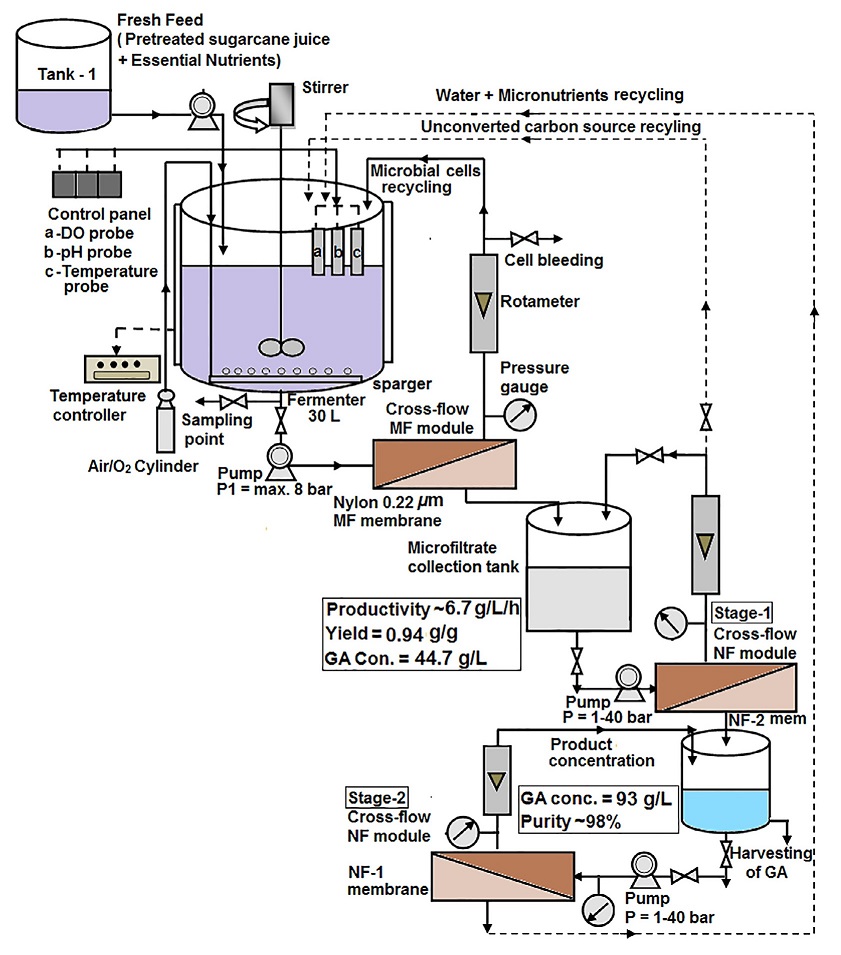
D-gluconic acid is commercially produced by biological fermentation using two main organisms: Aspergillus niger and Gluconobacter suboxydans.
3.4.1. Aspergillus niger process
Glucose is oxidized to gluconic acid by the enzyme glucose oxidase in the fungal cells. This enzyme uses flavine adenine dinucleotide as a cofactor, ultimately producing hydrogen peroxide. Catalase, another enzyme present in the fungus, breaks down the hydrogen peroxide into water and oxygen.
The gluconic acid formed can exist in equilibrium with its lactone form. The optimal pH for this process is around 5.6.
Large-scale production involves fermenting a sterilized nutrient broth containing glucose, magnesium sulfate, potassium phosphate, and a nitrogen source. The broth is inoculated with a culture of Aspergillus niger.
During fermentation, the activity of glucose oxidase increases rapidly, leading to a corresponding rise in gluconic acid production. The process typically runs for 40–100 hours, and maintaining a good oxygen supply and proper pH control are important for efficient yield.
After fermentation, the fungal biomass is removed by filtration, and the gluconic acid solution is purified by various steps like decolorization and filtration. Depending on the desired end product, the solution can be crystallized to obtain gluconic acid or spray-dried to yield a concentrated solution.
3.4.2. Gluconobacter suboxydans process
This process uses two glucose dehydrogenase enzymes for the conversion of glucose to gluconic acid. Unlike the Aspergillus niger method, Gluconobacter suboxydans requires less aeration due to its higher oxygen affinity.
Additionally, this organism tolerates more acidic conditions, allowing for the direct isolation of free gluconic acid from the fermentation broth.
3.4.3. Other methods and downstream processing
While not yet commercially viable, some processes using acidophilic methylotropic bacteria like Acetobacter methanolicus are being explored for direct gluconic acid production. Research is also ongoing on utilizing immobilized enzymes or microorganisms for this purpose.
Recovering free gluconic acid from fermentation broths that contain sodium gluconate (the sodium salt of gluconic acid) can be achieved by techniques like cation exchange or electrodialysis.
Crystallization from a supersaturated solution is the primary method for obtaining pure gluconic acid in its lactone form. Dehydration with specific alcohols followed by crystallization is another method for lactone production.
4. Uses of Gluconic Acid
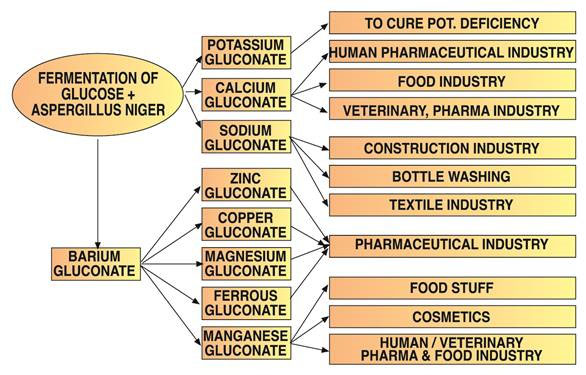
Gluconic acid is used for cleaning applications because of its ability to dissolve metal oxides, hydroxides, and carbonates and also because of the formation of water-soluble complexes with these cations.
Gluconic acid is used to remove calcareous and rust deposits from various metals, including galvanized iron, magnesium alloys, and stainless steel. It is employed in cleaning beer and milk scale from these surfaces.
In conjunction with magnesium salts, gluconic acid acts as a stabilizer for peroxide bleach baths in the textile industry.
D-gluconic acid’s physiological properties make it suitable for food and beverage applications. Low concentrations (0.02-0.1%) effectively invert sucrose without further fructose reactions.
Trace elements are often administered as gluconate salts due to their high bioavailability and tolerance. Potassium gluconate, available in anhydrous or monohydrate forms, has specific pharmaceutical uses.
Gluconic acid 1,5-lactone is a convenient substitute for the free acid in many examples. It offers advantages in acidic environments, such as pickling goods, curing sausages, and leavening baked goods.
Sodium gluconate is the most common gluconate salt. It forms complexes with metal cations, with stability increasing at higher pH. It effectively cleans various surfaces and removes grease, corrosion, rust, and oxide coatings from aluminum, steel, copper, and their alloys.
Hot (95–100 °C) alkaline sodium gluconate solutions efficiently remove paint and varnish without damaging underlying surfaces.
Gluconate solutions are used in pretreating surfaces for nickel-cobalt brazing on aluminum and preparing smooth, shiny nickel, tin, and zinc electroplated surfaces. It is increasingly replacing the toxic cyanide ion in some applications.
Sodium gluconate, sometimes combined with polyphosphates, is used in the paper industry as a sizing agent that imparts acid resistance to paper. In textiles, it aids in desizing polyester or polyamide fabrics and finishing natural cellulose fibers.
Sodium gluconate’s stability at high temperatures and pH, coupled with its ability to sequester water-hardening agents, makes it a useful component of commercial cleaners. It is used in bottle washing and cleaning aluminum surfaces.
Concrete manufacturers utilize sodium gluconate as a highly effective curing retardant. It improves concrete homogeneity, water resistance, frost resistance, and workability while reducing cracking.
A significant advantage of gluconic acid, its lactone, and their salts is their biodegradability in wastewater treatment systems. This extends to their metal complexes with aluminum, copper, iron, and zinc. Even chromium gluconate complexes biodegrade, albeit slower.
Released metal ions are removed during wastewater purification, reducing the potential mobilization of heavy metals. Biodegradation and hydroxide precipitation (at pH 9–10) contribute to the destruction of heavy metal gluconate complexes in wastewater.
References
- Gluconic Acid; Ullmann’s Encyclopedia of Industrial Chemistry. – https://onlinelibrary.wiley.com/doi/10.1002/14356007.a12_449
- S. Banerjee et al., Fermentative production of gluconic acid: A membrane-integrated Green process, Journal of the Taiwan Institute of Chemical Engineers (2018), https://doi.org/10.1016/j.jtice.2018.01.030