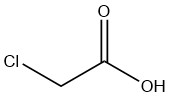
Pure chloroacetic acid is a colorless, hygroscopic crystalline solid that exists in various crystalline forms. It is a synthetic compound that was first discovered as a chlorination product of acetic acid by N. LEBLANC in 1841. R. HOFFMANN later synthesized it in 1857 by initiating the chlorination of acetic acid using sunlight.
Chloroacetic acid and its sodium salt are considered the most significant chlorination products of acetic acid in terms of industrial and economic importance.
Table of Contents
1. Properties of Chloroacetic acid
Chloroacetic acid has excellent solubility in water and good solubility in methanol, acetone, diethyl ether, and ethanol, but is only sparingly soluble in hydrocarbons and chlorinated hydrocarbons.
1.1. Chemical Reactions of Chloroacetic acid
The chemical properties of chloroacetic acid can be attributed to the high reactivity of its carboxylic acid group and the ease of substitution of its α-chlorine atom.
Chloroacetic acid and its derivatives, such as salts, esters, anhydrides, acyl chlorides, amides, and hydrazides, are widely used as synthetic organic intermediates.
Industrial applications of chloroacetic acid include reactions with inorganic and organic bases, which yield salts. Some salts can also form adducts with chloroacetic acid, and sodium chloroacetate is an important commercial product.
Chloroacetic acid esters, such as methyl, ethyl, and tert-butyl chloroacetate, are synthesized by reacting with alcohols or olefins.
Chloroacetyl chloride is produced from chloroacetic acid by reacting with various reagents such as POCl3, PCl3, PCl5, thionyl chloride (SOCl2), or phosgene (COCl2).
The acid can also react with chloroacetyl chloride to produce bis(chloroacetic)anhydride, which can be obtained by dehydration of chloroacetic acid with P2O5 or by reacting with acetic anhydride.
Chloroacetyl chloride forms mixed anhydrides with other carboxylic acids, such as acetic chloroacetic anhydride.
Nucleophilic substitution of the chlorine atom is an important reaction when chloroacetic acid is used as an intermediate in organic syntheses.
For instance, hydrolyzing neutral or basic aqueous solutions of chloroacetic acid yields glycolic acid (hydroxyacetic acid) and diglycolic acid (2,2′-oxydiacetic acid), an industrial method of producing these compounds.
Heating the salts of chloroacetic acid gives glycolide, 1,4-dioxine-2,5-dione. Reaction with sodium or potassium hydrogensulfide forms thioglycolic acid and thiodiglycolic acid.
Chloroacetic acid also reacts with ammonia, producing aminoacetic acid (glycine) as the main product or nitrilotriacetic acid, depending on the reaction conditions.
When methyl chloroacetate reacts with ammonia at low temperature, chloroacetamide is obtained. By reacting with tertiary amines in alkaline solutions, various commercially important betaines are formed, such as N-lauryl betaine.
Furthermore, aromatic compounds like naphthalene undergo electrophilic substitution with chloroacetic acid over suitable catalysts to form arylacetic acids.
The commercially important cyanoacetic acid, which is used as an intermediate in the production of synthetic caffeine, is synthesized by reacting potassium cyanide with chloroacetic acid in a neutral solution.
Iodoacetic acid is formed by reacting with potassium iodide, while phenoxyacetic acids are synthesized by phenol etherification in the presence of sodium hydroxide.
Additionally, carboxy-methyl derivatives with a relatively high degree of etherification are synthesized by reacting polysaccharides, such as cellulose, starch, guar, etc., in a strongly alkaline sodium hydroxide medium.
2. Production of Chloroacetic acid
Numerous techniques have been suggested and patented for the manufacturing of chloroacetic acid. In the past, the industrial production of chloroacetic acid involved the hydrolysis of 1,1,2-trichloroethylene with sulfuric acid as a catalyst (1) and the chlorination of acetic acid with chlorine as a catalyst (2). However, only the latter and more older process is currently employed for the production of chloroacetic acid on an industrial scale.
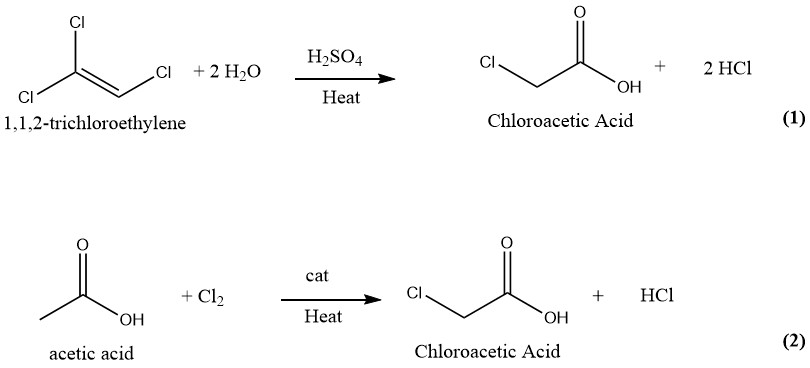
2.1. Hydrolysis of Trichloroethylene
Chloroacetic acid can be produced using a continuous process in which equal amounts of trichloroethylene and 75% sulfuric acid are reacted at 130-140°C. Upon complete conversion of trichloroethylene, the reaction mixture contains about 50% chloroacetic acid and 1–2% water.
Subsequently, the blend is subjected to vacuum distillation to obtain pure chloroacetic acid. During this process, the vapors are washed with water, which is subsequently reused as a diluent for sulfuric acid.
The hydrogen chloride gas generated during the process is washed with fresh trichloroethylene and then purified by freezing and absorption in water.
This method yields 1000 kg of finished product and 700–750 kg of HCl gas as a byproduct from 1500–1850 kg of trichloroethylene and 600 kg of H2SO4 (95%).
The trichloroethylene method of producing chloroacetic acid produces highly pure chloroacetic acid that is free of di- or trichloroacetic acid. However, this method is no longer in use due to the high cost of trichloroethylene and the significant amount of HCl generated as a byproduct.
The purification procedure involves the separation of chloroacetic acid from trichloroethylene, sulfuric acid, and water.
2.2. Chlorination of Acetic Acid
2.2.1. Synthesis
Highly selective conversion of acetic acid into chloroacetic acid is achievable by chlorination of acetic acid that employs appropriate catalysts. The reaction uses acetic anhydride as a catalyst, which facilitates the following reaction mechanism:
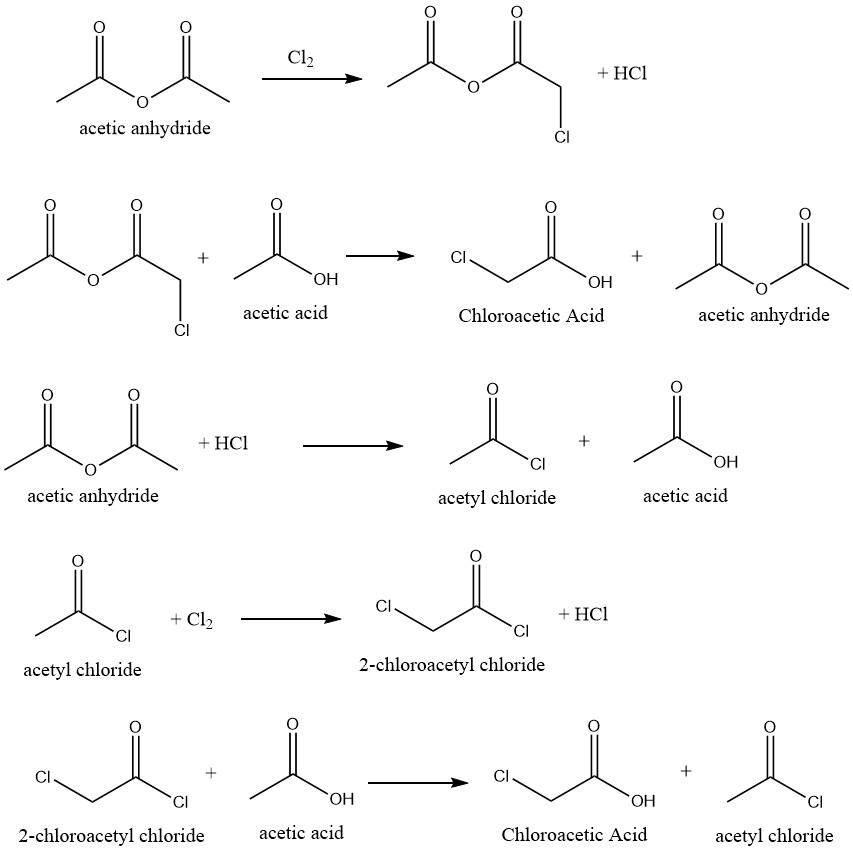
Several inhibitors have been suggested to hinder the formation of dichloroacetic acid, which is generated by the chlorination of chloroacetic acid in the crude mixture. By doing so, it eliminates or decreases the requirement for the purification process to produce technical-grade products.
2.2.2. Purification
To achieve the required level of purity for many products, separation of di- and trichloroacetic acids is necessary. However, the close boiling points of these chlorinated acetic acids render fractional distillation impractical.
Alternative techniques, such as azeotropic distillation and extractive distillation, have been suggested for dichloroacetic acid separation, but their effectiveness remains uncertain.
A significant industrial purification process involves solvent-free crystallization, which is based on the α-modification of chloroacetic acid’s higher melting point. The di- and trichloroacetic acids are removed from the mother liquor after crystallization.
Crystallization can occur in stationary finger crystallizers or agitated stirrer crystallizers. A centrifuge is used to separate the mother liquor from the crystal slurry after crystallization in the latter.
The product is then washed with water or acetic acid. The pure chloroacetic acid is commonly melted and transformed into flakes. In stationary machines, crystallization is carried out by using cold fingers.
When all the chloroacetic acid has crystallized, the mother liquor is drained, and the pure crystalline product is melted and flaked.
A thin-layer crystallization process with raw materials has been proposed as an alternative purification method.
Additionally, solvent-based crystallization processes using carbon tetrachloride, dichloromethane, or hydrocarbons with three chlorine atoms have been reported, resulting in easily filterable crystals.
Regardless of the method used, a mother liquor consisting of acetic acid, chloroacetic acid, and di- and trichloroacetic acids is generated. In ideal conditions, this mixture can be further chlorinated to yield the useful trichloroacetic acid.
A more significant purification method involves catalytic hydrodechlorination of undesired byproducts, such as dichloro- and trichloroacetic acid. Di- and trichloroacetic acids can be dechlorinated through catalytic hydrogenation at high temperature, typically using palladium on a carrier (e.g., carbon or silica gel).
When vapor phase catalytic hydrodechlorination is used, dichloroacetic acid is mainly converted to acetic acid. However, the use of finely dispersed, inert carriers with Pd in the liquid phase at 130–150°C results in selective dechlorination to form chloroacetic acid.
Modifications of this procedure, such as spraying crude acid with hydrogen gas under vacuum or trickling acid over the catalyst in a fixed bed, have also been proposed. The addition of HCl to the crude acid before contact with the catalyst in the fixed bed enhances selectivity and saponifies acid chlorides and anhydrides prior to dechlorination.
The Pd catalyst on a silica gel carrier (40–200 mm particle size) is highly active and selective, and co-catalytically effective additives, such as sodium acetate, can further improve results.
Surface enrichment of the noble metal enhances catalyst efficiency, and spent catalyst can be reactivated via chlorine treatment.
Using Pd catalysts on optimized active charcoal carriers reduces over-reduction byproducts (e.g., aldehydes) during the hydrogenation step, which can be performed using a loop reactor.
A method to decrease the concentration of dichloroacetic acid in chloroacetic acid from 2000 to 210 ppm can be accomplished by using non-noble metal catalysts at 100 to 140°C without hydrogen.
Figure 1 shows an industrial chlorination process, where a mixture of acetic acid, acetic anhydride, and recycled acetyl chloride is chlorinated in reactor (a) at 90–140°C. The HCl gas formed contains only trace amounts of chlorine.
The resulting mixture of chloroacetic acid, acetic acid, and acetic anhydride is condensed using water-cooled condensers (b) and returned to the reactor. Acetyl chloride entrained in the HCl gas is recovered in a subsequent low-temperature process (c) and recycled.
The HCl gas is purified and converted into concentrated aqueous hydrochloric acid.
The crude acetic acids can be vacuum distilled (d) prior to the dechlorination step, which is carried out at 120–150°C using a palladium catalyst and a large excess of hydrogen. Acetic acid is taken overhead from the vacuum fractionation column (i), while the bottom product is pure chloroacetic acid.
If desired, the chloroacetic acid can be further purified by distillation to remove high-boiling impurities, such as the condensation products of aldehydes formed during the hydrogenation step.
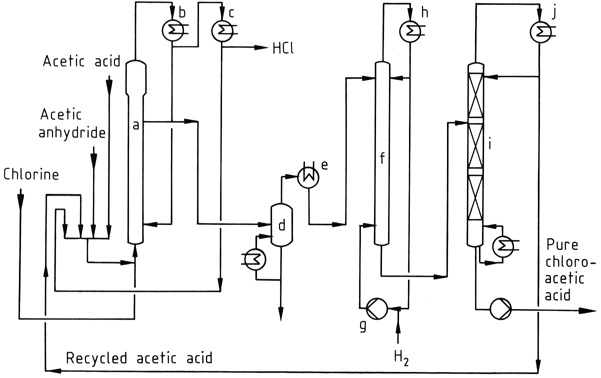
a) Chlorinating reactor; b) Condenser for acetic acid, acetic anhydride, chloroacetic acid; c) Condenser for acetyl chloride; d) Evaporator; e) Condenser for chloroacetic acid; f) Hydrogenation reactor; g) Hydrogen compressor; h) Condenser; i) Distillation column; j) Condenser for acetic acid
3. Uses of Chloroacetic Acid
Chloroacetic acid is a versatile chemical compound that is utilized in a wide range of industrial applications. One of its primary uses is in the manufacture of carboxymethyl cellulose, or CMC, which amounts to several hundred thousand tons annually.
In addition to CMC, chloroacetic acid can also react with starch to produce carboxymethyl starch, which is as widely used as CMC. While other polysaccharides can also be modified with chloroacetic acid, they are not as commonly employed.
Another significant application of chloroacetic acid is in the production of herbicides based on arylhydroxyacetic acids, specifically Chlorophenoxyalkanoic Acids. These herbicides are among the most widely used in the agricultural sector.
In addition, chloroacetic acid and methyl chloroacetate are used in the production of the insecticide dimethoate, as well as the herbicides benazoline and methyl b-naphthyloxyacetate.
A third important use of chloroacetic acid is in the manufacture of thioglycolic acid, also known as mercaptoacetic acid. This is achieved by reacting chloroacetic acid with sodium or potassium hydrogen sulfide, or other sulfur compounds.
Thioglycolic acid is employed in various forms, including its salt, ester, or derivative. The majority of thioglycolic acid produced is used in the production of stabilizers for poly(vinyl chloride).
It is also used in hair cosmetics as well as in other industrial applications such as the production of long-chain betaines, such as N-lauryl betaine, which are surfactants used in cleaners and personal care products.
In addition to these applications, chloroacetic acid has a multitude of other uses in organic synthetic reactions. For instance, cyanoacetic acid or its esters can be employed to manufacture caffeine and barbiturates, which are important hypnotics.
Chloroacetic acid can condense with aromatic hydrocarbons to form arylacetic acids.
When reacted with naphthalene, 1-naphthylacetic acid is produced as the main product, and 2-naphthylacetic acid is generated as the byproduct. Both substances are known to promote plant growth.
Lastly, chloroacetic acid plays an essential role in the synthesis of coumarin and vitamin B6.
Reference
- Chloroacetic Acids; Ullmann’s Encyclopedia of Industrial Chemistry. – https://onlinelibrary.wiley.com/doi/10.1002/14356007.a06_537.pub3