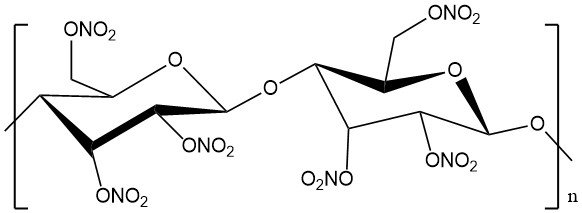
Cellulose nitrate, also known as nitrocellulose, flash paper, guncotton, or celluloid, is a chemical product derived from the natural polymer cellulose. It is a white, odorless, and tasteless substance. Its characteristics are dependent on the degree of substitution.
Table of Contents
1. Physical Properties of Cellulose Nitrate
The density depends heavily on the degree of nitration; it increases with increasing the degree of substitution. Commercially available, moistened cellulose nitrate cotton types exhibit bulk densities within 0.25-0.60 kg/L, decreasing to 0.15-0.40 kg/L (dry mass).
Cellulose nitrate chips containing at least 18% dibutyl phthalate reach a density of 1.45 g/cm³, with a bulk density of 0.3-0.65 kg/L.
Fineness significantly impacts the specific surface. The Rossin apparatus provides accurate measurements, revealing values between 1850 and 4700 cm²/g. Inner surface area, determined via the BET method, exhibits dependence on molar mass. However, lowering the degassing temperature due to cellulose nitrate’s flammability raises uncertainty about complete desorption.
Thermodynamic Properties: Table 1 summarizes the most important values.
Property | Value |
---|---|
Heat of combustion | 12 kJ/g |
Heat of fusion | 15 J/g |
Specific heat capacity | 1.5 J/(g·K) |
Thermal conductivity | 0.2 W/(m·K) |
Glass transition temperature | 50-60 °C |
Decomposition temperature | 180 °C |
Electrical Properties: Measured on celluloid (30 wt% camphor in cellulose nitrate), are listed below:
Dielectric constant:
- at 50-60 Hz: 7.0-7.5
- at 106 Hz: 6.0-6.5
Dissipation factor (tan δ):
- at 50–60 Hz: 0.09–0.12
- at 106 Hz: 0.06-0.09
Specific resistance: 1011–1012 Ω⋅cm
The stress-strain curve reveals the dependence of elongation and tensile strength on molecular size (expressed as viscosity). A higher molecular mass translates to more elastic films with increased tensile strength.
Optical Properties: The crystalline structure renders cellulose nitrate films optically anisotropic. Colors in polarized light shift with nitrogen content:
- 11.4% N: weakly red
- 11.5-11.8% N: yellow
- 12.0-12.6% N: blue to green
The index of refraction is 1.51, with peak light transmission at 313 nm.
Light Stability: Sunlight, particularly ultraviolet radiation, discolors and embrittles cellulose nitrate films. Solvents, plasticizers, and resins can influence the extent of yellowing.
2. Chemical Properties of Nitrocellulose
Cellulose nitrate is produced by partial or complete nitration of the cellulose’s three hydroxyl groups and exhibits varying nitrogen content based on the degree of nitration.
- Cellulose mononitrate: 6.75% N
- Cellulose dinitrate: 11.11% N
- Cellulose trinitrate: 14.14% N
Nitrocellulose with 10.8–12.6% N is suitable for lacquers due to its solubility in organic solvents, and above 12.3% N, it is primarily used in explosives.
The nitrogen content directly relates to the degree of substitution, determining solubility in organic solvents.
- Alcohol-soluble nitrocellulose (A types): ~10.9–11.3% N (highly soluble in alcohols, esters, and ketones)
- Moderately soluble nitrocellulose (AM types): ~11.4–11.7% N (soluble in esters, ketones, glycol ethers, and excellent solubility with alcohols)
- Cellulose nitrate soluble in esters (E types): 11.8–12.2% for lacquer cotton, up to 13.7% for guncotton (readily soluble in esters, ketones, and glycol ethers).
Intrinsic viscosity reflects the average molecular length (degree of polymerization) and can be mathematically linked to molecular mass via the Staudinger-Mark-Houwink equation.
Polymolecular data, including degree of polymerization and molecular mass distribution, complement viscosity, and solubility, are important for understanding mechanical properties and aging processes. Gel permeation chromatography efficiently isolates polymers based on their molecular mass.
Cellulose nitrate is very compatible with numerous substances found in nitro lacquers, such as alkyd resins, maleic resins, ketone resins, urea resins, polyacrylates, and various softeners.
Strong acids, bases, and organic amines can rapidly degrade or decompose cellulose nitrate, potentially leading to deflagration. The ester bonds contribute to its inherent instability, which is influenced by temperature, sample form, and the presence or absence of decomposition products.
Mixed sulfuric acid esters, introduced during production, impart further instability, requiring a stabilization step with weakly acidic water.
Above 100 °C, cellulose nitrate undergoes thermal decomposition via a series of exothermic oxidation reactions initiated by NO2 radicals, potentially leading to spontaneous deflagration.
Stabilizers like diphenylamine or weak acids can bind intermediary nitric oxides and prevent this autocatalytic chain reaction. Deflagration temperature, influenced by stabilization and plasticization levels, serves as a key measure of thermal stability.
The Bergmann-Junk test and warm storage test provide additional methods for evaluating the stability of cellulose nitrate.
3. Nitrocellulose Raw Materials
The raw materials used in the production of cellulose nitrate are cellulose and nitrating agents.
3.1. Cellulose
Prior to World War I, only high-purity cotton linters (bleached flakes or crape) were used for nitration due to their superior yield and clarity.
The scarcity of linters led to experimentation with other sources like wood cellulose, even unbleached, and annual plant fibers. However, these resulted in inferior lacquers with dullness and poor mechanical properties due to the high pentosan content.
However, the advancements in chemical-grade wood pulp purification with hot or cold alkali treatments (R18 values > 92%) enabled its use for nitration similar to linters. Highly refined prehydrolyzed sulfate pulps (R18 > 96%) are particularly suitable and allow viscosity control through initial cellulose choice.
Low ash and calcium content in cellulose is important in preventing calcium sulfate precipitation during industrial nitration.
Studies show that the morphological and chemical properties of cellulose, along with the presence of hemicellulose and lignin, influence nitrating capability and final product properties. Linters exhibit superior acid retention capacity (110–130%) compared to wood pulp (up to 300%), impacting fiber mass retention during processing.
A specialized machine measures fiber pile compression and relaxation to determine if a raw material is suitable for nitration.
Approximately 3.4% (150,000 tons) of global chemical-grade pulp production is used for cellulose nitrate manufacturing.
3.2. Nitrating Agents
Schöenbein’s nitric/sulfuric acid/water system remains the preferred industrial nitrating agent. The maximum achievable degree of substitution (DS 2.7, 13.4% N) requires anhydrous nitric acid and a 1:2 molar ratio of HNO3 to H2SO4 monohydrate.
The optimal nitrating mixture contains 21.36% HNO3, 66.44% H2SO4, and 12.20% H2O.
Water content significantly impacts achievable nitration, with a drop in yield above 12%. Desired esterification levels are adjusted by varying the nitrating acid mixture (Table 2), with industrial processes maintaining HNO3 at 25–26%.
Nitrocellulose type | % HNO3 | % H2SO4 | % H2O | N content, % | DS |
---|---|---|---|---|---|
Lacquer cotton A | 25 | 55.7 | 19.3 | 10.75 | 1.90 |
Celluloid cotton | 25 | 55.8 | 19.2 | 10.90 | 1.95 |
Lacquer cotton AM | 25 | 56.6 | 18.4 | 11.30 | 2.05 |
Dynamite cotton | 25 | 59.0 | 16.0 | 12.10 | 2.30 |
Lacquer cotton E | 25 | 59.5 | 15.5 | 12.30 | 2.35 |
Powder cotton | 25 | 59.8 | 15.2 | 12.60 | 2.45 |
Gun cotton | 25 | 66.5 | 8.5 | 13.40 | 2.70 |
The ternary system HNO3/H2SO4/H2O has been extensively studied, with the phase diagram revealing three distinct zones:
- Technical nitration zone: Suitable for nitration (10-100% nitric acid, 0-80% sulfuric acid, 0-20% water).
- Solution zone: No significant nitration; cellulose degradation occurs (0–10% nitric acid, 60–100% sulfuric acid, 0–40% water).
- Swelling zone: Increased water content leads to decreased nitration (nitric acid content varies, sulfuric acid 60–100%, water increases).
A less common process uses magnesium nitrate instead of sulfuric acid as a dehydrating agent, offering potential for continuous processing and waste reduction.
4. Production of Cellulose Nitrate
Cellulose nitrate is produced by treating cellulose, usually cotton linters, with a mixture of concentrated nitric and sulfuric acids. The degree of nitration is controlled by adjusting the temperature, acid concentrations, and reaction time.

The flow diagram (Figure 1) shows the industrial production of nitrocellulose by the mixed acid process. The viscosity of the final product is determined by the choice of the initial cellulose, and the degree of nitration is determined by the composition of the mixing acid. The final viscosity is adjusted during the pressure-boiling step.
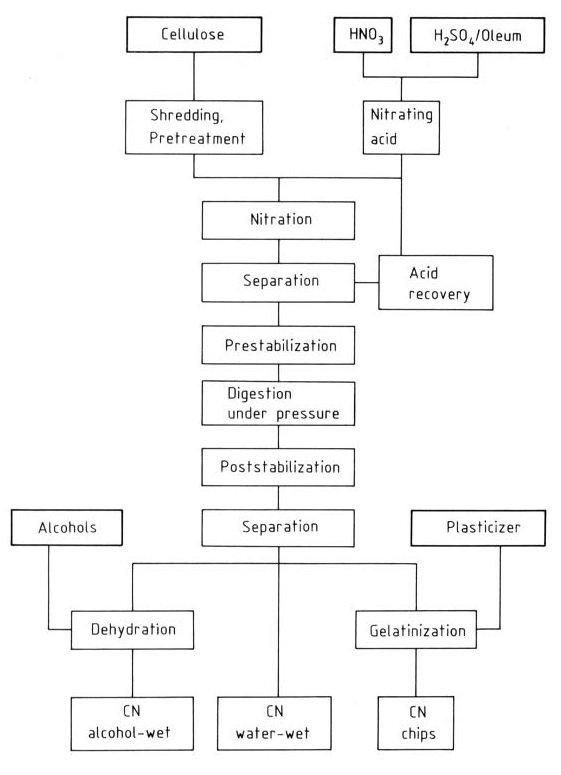
4.1. Cellulose Preparation
The processing of cellulose plays an important role in achieving rapid, uniform nitration. Traditionally, cotton linters with low moisture content (<7%) were mechanically disintegrated to obtain a homogeneous fiber mass. However, this process was laborious and limited in scale.
Pressed pulp sheets offered an alternative but required shredding into small pieces to facilitate efficient nitration. Spruce or beech cellulose pre-treated with 20% sodium hydroxide (mercerization) was historically used for this purpose, often in the form of lightweight crape paper (25 g/m³).
Seeking to avoid the costly paper sheet conversion, attempts were made to directly disintegrate pulps into loose, linter-like fibers. A 50% moisture content proved optimal for nitration and subsequent acid washout but required drying before nitration, adding another processing step.
The Stern shredder represented a significant advancement by tearing pulp sheets instead of cutting, minimizing fiber compression and improving nitration uniformity.
Modern cellulose for nitration is primarily used in the form of fluff, shreds, or chips. The packing density and compression behavior of these forms significantly impact the swelling and nitration kinetics, as well as the acid retention capacity. Optimizing these factors is crucial for efficient and controlled nitration processes.
4.2 Nitration of Cellulose
The dominant industrial process for cellulose nitration is still a batch system developed in 1922 by DuPont. The equipment is made of stainless steel. The preheated nitrating acid is added to the stirring reactor containing cellulose.
A large acid excess (1:20 to 1:50) ensures stirrability and heat removal. Nitration temperature ranges from 10 °C (dynamite) to 36 °C (celluloid).
After 5 minutes, the reaction is complete, and the mixture remains in the reactor for 30 minutes due to temperature sensitivity. Hydrolytic degradation with significant yield losses begins above 40 °C.
Theoretical yields for common nitrocellulose types (DS 1.8–2.7, N content 10.4–13.4%) reach 150–176% based on cellulose, but practical yields are 15% lower due to cellulose type and purity, temperature, and duration.
Losses arise from complete cellulose decomposition to oxalic acid by oxidation of oligosaccharides and monosaccharides with nitric acid. Mechanical losses during subsequent separation (especially short hardwood fibers) also contribute.
The reaction mixture is centrifuged at high speed to separate and recycle excess acid. Maintaining moisture prevents ignition or deflagration.
Acid retention by the product is important for economic reasons. Linters (100–130% retention) significantly outperform wood celluloses (up to a 3x higher cellulose nitrate-to-acid ratio), depending on wood or cellulose type and processing.
The acid-moist product is immediately diluted in excess water (1% consistency) to rapidly displace clinging acid and prevent cellulose nitrate saponification.
Developed in the 1960s, continuous nitration processes offer economic advantages, uniform product quality, and improved safety. The system employs two or more cascade-connected straight-run tanks or tubes equipped with conveyors (screw or turbulence mixers) to advance the reaction mixture.
Prepared cellulose continuously mixes with added nitrating acid in this cascading system. It is important that the cellulose is rapidly added and immediately covered with acid. Residence time is 30-55 minutes, but a newer loop-formed pressure reactor needs only 6–12 minutes.
The reactant then enters a continuous centrifuge for simultaneous excess acid separation and water dilution. The short residence time (only a few seconds) minimizes deflagration and saponification risks.
Figure 2 illustrates the schematic of the Hercules continuous process.
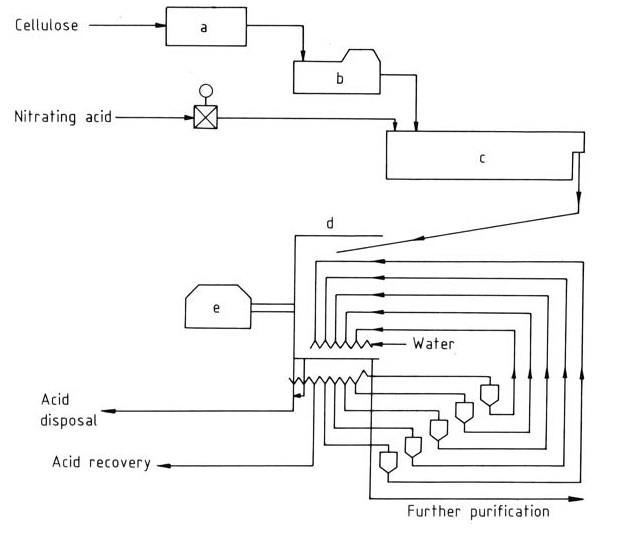
a) Preconditioning; b) Auto-matic scale; c) Reactor; d) Washing zone; e) Centrifuge
Preconditioned cellulose enters the continuous reactor via automatic scales, and then the reaction product is centrifuged and countercurrently washed in water zones. The nearly acid-free product exits the centrifuge, while the washed acid can be recycled with minimal loss.
4.3. Stabilization and Viscosity Adjustment of Nitrocellulose
Following prewashing, repeated washing and boiling with water containing 0.5–1% acid residue further purifies the cellulose nitrate. This batch method requires significant space, water, and energy, with boiling times varying between 6 hours for celluloid and 40 hours for guncotton. Automated continuous processes have also been developed.
Prestabilization removes most of the remaining sulfuric acid, which catalyzes cellulose nitrate decomposition. The acid binds through adsorption and esterification. Weakly nitrated cellulose nitrate retains 1-3% total sulfate, with 70–85% as acidic semiesters.
Highly nitrated cellulose nitrate has only 0.2–0.5% sulfate, of which 15–40% may be esters. Semiesters are easily saponified and washed out by boiling water. The existence of “resistant” sulfate as a neutral ester or adsorbed acid remains unclear.
Pressure boiling (digestion) at 6-8% consistency and 130–150 °C adjust the final cellulose nitrate viscosity through controlled polymer degradation. The remaining trace of sulfuric acid promotes hydrolysis at this temperature and pressure. For example, a 3-hour treatment at 132 °C can reduce viscosity to 1/10th of its initial value.
This process facilitated the development of high-solids coatings and protective nitro lacquers. Guncotton stabilization benefits from pressure boiling, while dynamite wools usually bypass it.
Chain degradation generates further product losses as soluble fragments and oxalic acid. Nitrous gases (NOx) from nitric acid reduction are continuously removed to prevent cellulose nitrate decomposition.
Pressure boiling can be performed batchwise in autoclaves or continuously in tubular reactors (e.g., 1500 m long, 100mm diameter) using direct steam. A one-pot process combining prestabilization, pressure boiling, and poststabilization in a single operation exists.
Additional washing and boiling nearly eliminate the remaining sulfuric acid. Celluloid and lacquer cellulose nitrate are finished in flaky or fibrous form, while guncotton requires grinding. This occurs in Hollander engines at 12–15% consistency or continuously in cone refiners, gradually concentrating the material from 3% to 10% across stages.
Hydrocyclones during the final washing facilitate sorting. Grinding aids diffusion, removing the last fiber-capillary acid residues against water flow. Weak bases (sodium carbonate or chalk) maintain a pH of 7, and stabilizers (organic acids) may be added.
5. Uses of Cellulose Nitrate
Cellulose nitrate is used in the production of explosives, lacquers, dispersions, and celluloid.
1. Explosives
Based on usage, explosives can be blasting agents, propellants, detonators, igniters, or pyrotechnics.
Cellulose nitrates are used in propellants and gunpowder and are categorized as:
- Monobasic: Purely based on cellulose nitrate.
- Dibasic: Contains additional energy carriers like nitroglycerin or diglycol dinitrate.
- Tribasic: Includes a third component like nitroguanidine, offering lower heat of combustion and extended gun barrel life.
Specific types of cellulose nitrate are used based on nitrogen content:
- CP I (Guncotton): contains 13.3–13.5% N, highly nitrated cellulose used for propellants.
- CP II (Collodium): contains 12.0-12.7% N and is primarily used for smokeless powder.
- PE (Powder Standard): contains 11.5–12.0% N, is less nitrated, and is used for smaller arms.
Aromatic amines like diphenylamine are used as stabilizers in gunpowder by binding nitrous gases generated during decomposition. Mixtures of CP I and CP II offer the desired energy content and can also be used.
Granules of cellulose nitrate used in gunpowder are coated with graphite to prevent electrostatic charges. Its main applications are in small-arms ammunition, large-caliber guns, and tanks.
2. Lacquers
The film-forming abilities of cellulose nitrate, achieved by physical drying, are the foundation of its diverse lacquer applications. It is compatible with a vast array of resins, softeners, pigments, and additives, allowing the creation of lacquers with specific properties.
Solvent mixtures play an important role in film formation, influencing drying time and other lacquer characteristics. Cellulose nitrate lacquers are industrially used in the wood, metal, paper, foil (including hot sealing), and leather industries, as well as in other applications like adhesives, putties, and printing inks.
Application techniques like spraying, casting, rolling, doctor knife coating, and dipping offer flexibility based on the substrate and desired outcome. Matching the viscosity and solubility of the chosen cellulose nitrate type to the specific application ensures optimal performance.
Finally, environmentally friendly aqueous dispersions with reduced organic solvents are available for certain applications, addressing sustainability concerns.
3. Dispersions
Traditional lacquers made with cellulose nitrate contain a lot of organic solvents (60–90%). These solvents are released into the air as the lacquer dries, contributing to air pollution and potential health risks. They are also expensive and may pose disposal challenges.
Aqueous cellulose nitrate dispersions replace the organic solvents with water. While they offer advantages, these dispersions present some challenges:
- Cellulose nitrate itself doesn’t dissolve in water, requiring the addition of special chemicals called coalescents to help it form a film when applied.
- Currently, aqueous dispersions are not as widely used or versatile as traditional solvent-based lacquers. They are best suited for specific materials like leather, wood, foil, and metal.
Examples of commercially available aqueous dispersions are Isoderm (Bayer AG), Coreal (BASF), and special types of Waloran N (Wolff Walsrode AG).
4. Celluloid
A special use of cellulose nitrate is in the production of celluloid, which is produced by mixing cellulose nitrate (10.5–11.0% N) with softeners like camphor and solvents in a kneader.
Celluloid has largely been replaced by synthetic materials but is still used for combs, hair ornaments, toilet articles, office supplies, ping-pong balls, and specific applications.
6. Toxicology of Cellulose Nitrate
Cellulose nitrate production involves hazardous chemicals requiring specific safety measures and regulations.
Precursor Chemicals
- Sulfuric acid: Concentrated acid (5-15% and above) falls under EC-No. 016-020-01-5 and 016-020-00-8, respectively, and is subjected to the Arbeitsstoffverordnung (working substance regulation) requiring proper labeling.
- Nitric acid: Similar to sulfuric acid, concentrated and mixed nitrating acids are classified under EC-No. 007-004-01-9 and 007-004-00-1, requiring labeling and adhering to the Arbeitsstoffverordnung.
Additionally, their oxidizing properties necessitate caution when handling with organic materials. MAK (maximum workplace concentration) values are set at 10 mL/m³ (ppm) for nitric acid vapors and 5 mL/m³ (ppm) for nitrogen oxides (NO2), prompting regular employee checkups for potential obstructive respiratory tract illnesses.
Cellulose Nitrate
Contrary to its precursors, cellulose nitrate itself is not inherently toxic or hazardous to health. However, potential health risks arise from:
- Damping agents: Inhalation of damping agents like cellulose nitrate and nitrous gases released during combustion or smoldering can be harmful.
- Phlegmatizers: While commercially available cellulose nitrate for lacquer production (nitrogen content < 12.6%) contains at least 18% gelatinizing softener, conflicting regulations exist.
The Arbeitsstoffverordnung classifies it as a hazardous substance requiring specific packaging and labeling, echoing similar EEC regulations (1982). However, damping agents like ethanol and 2-propanol are not subject to these regulations, and butanol only falls under category II d with no health concerns when present in damped mixtures at a maximum concentration of 35%.
Reference
- Cellulose Esters; Ullmann’s Encyclopedia of Industrial Chemistry. – https://onlinelibrary.wiley.com/doi/10.1002/14356007.a05_419.pub2