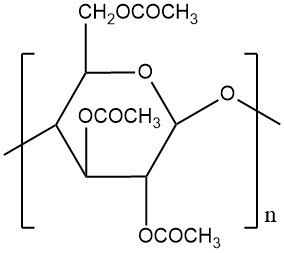
Cellulose acetate is a commercially important thermoplastic, formed from the acetylation of cellulose (a natural polymer). This reaction converts all primary and secondary hydroxyl groups within the cellulose chain into cellulose triacetate, a fully esterified intermediate, which can be isolated or further processed.
Following acetylation, a controlled hydrolysis step regenerates a desired proportion of hydroxyl groups, customizing the degree of acetyl substitution (DS) per glucose unit on the cellulose structure. DS, expressed as the degree of esterification or substitution, ranges from 0 to the theoretical maximum of 3.
Additional chemical alterations during cellulose acetate preparation include sulfation and desulfation of hydroxyl groups, as well as controlled chain scission, all facilitated by the catalytic action of the predominant acetylation catalyst, sulfuric acid.
Table of Contents
Several potential acetylation reagents exist, including acetic acid, acetyl chloride, ketene, and acetic anhydride. Acetic acid exhibits sluggish reactivity, resulting in products with very low acetyl content. Acetyl chloride, while investigated in combination with catalysts, lacks commercial viability.
Ketene, despite producing no byproducts, hasn’t found success as a commercially effective agent. As a result, acetic anhydride is the most effective, reacting with three hydroxyl groups per glucose unit to yield three molecules of acetic acid as a byproduct.
The initial application of acetic anhydride in 1869 involved a direct reaction with cellulose at 180 °C, likely causing significant product degradation. The discovery of catalytic benefits by Franchimont in 1879 facilitated lower-temperature processing with reduced degradation.
Numerous acetylation catalysts have been identified, but sulfuric acid is commercially important. Perchloric acid, while used commercially, causes equipment corrosion risks and safety concerns due to the instability of its neutralized salts.
Other mineral acids lack sufficient acidity in the typical acetic acid-acetic anhydride medium to be effective. Zinc chloride, once used commercially, has been abandoned due to high dosage requirements and recovery costs.
One key advantage of sulfuric acid lies in its rapid absorption onto the cellulose fiber surface during pretreatment, prior to acetic anhydride addition. This swelling and uniform catalyst distribution enhance the subsequent reactivity of the cellulose mass.
Importantly, sulfuric acid also catalyzes controlled chain scission through the hydrolysis of glycosidic linkages, achieving the desired reductions in molecular weight.
Upon adding acetic anhydride in stoichiometric excess to the pretreated cellulose, sulfuric acid forms a cellulose sulfate ester acid intermediate. Additionally, sulfuric acid reacts with acetic anhydride to produce acetyl sulfuric acid, both likely playing important roles in the esterification reaction.
The cellulose sulfate ester acid intermediate then reacts with the surrounding acetic acid-acetic anhydride medium, replacing the sulfate ester group with acetyl. This exothermic reaction must be balanced with the rate of catalyzed chain scission (acetolysis) to achieve the desired product molecular weight.
Hydrolysis of the acetyl groups, controlled by sulfuric acid and water concentration, occurs after complete esterification. The desired degree of acetyl substitution is reached by adjusting the water content and hydrolysis time. Finally, the catalyst is neutralized with an acetate salt to end the process.
It is noteworthy that a portion of the sulfate groups introduced during pretreatment remains bound to the cellulose as a combined sulfate ester even after acetylation. However, these groups are largely hydrolyzed during the subsequent water addition step, further contributing to controlled chain scission and customizing the final material properties.
1. Cellulose Acetate Feedstocks
The production of cellulose acetate is performed by reacting two primary raw materials: high-purity cellulose and acetic anhydride. Achieving optimal post-processing performance depends on the selection of these crucial ingredients.
1.1. Cellulose
Cotton linters offer a premium feedstock with a purity exceeding 99% α-cellulose. Following the removal of spinnable cotton fibers, two additional linter cuts are made. The first cut (4%), primarily used for medical cotton and felt, yields longer fibers. The second cut (8%), richer in shorter, layered fibers, is ideal for chemical processing.
Raw linters undergo rigorous purification: mechanical cleaning, boiling in dilute sodium hydroxide, and acid-alkaline bleaching. Controlled drying is crucial, as local over-drying (beyond the 3-8% moisture window) significantly dampens reactivity. Table 1 summarizes typical analytical values for high-quality linters.
Component | Content |
---|---|
α-Cellulose | 99.7 % |
β-Cellulose | 0.2 % |
γ-Cellulose | 0.1 % |
Carboxyl groups | <0.02 % |
Total ash | 0.02 % |
Degree of polymerization | 1000 – 7000 |
Wood pulp is the dominant feedstock and encompasses both softwood and hardwood sources. Historically, limited α-cellulose content (90–95%) restricted wood pulp usage due to lower-quality acetate production.
However, advances in sulfite and Kraft pulping techniques have enabled efficient lignin and hemicellulose removal, raising α-cellulose content above 95%.
Since the 1950s, the cost-effectiveness of higher-purity wood pulp has steadily displaced cotton linters. Remarkably, wood pulp-based cellulose acetates exhibit comparable tensile strength, color, solution clarity, and light/thermal stability to their linter counterparts.
1.2. Acetic Anhydride
Most manufacturers opt for on-site conversion of byproduct acetic acid to acetic anhydride, adjusting concentrations (90–95%) to suit their specific processes.
2. Industrial Production Processes of Cellulose Acetate
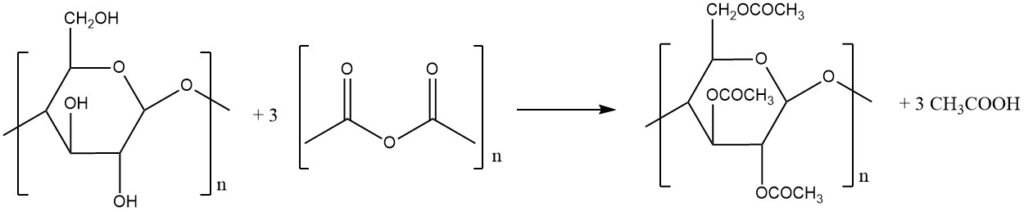
While numerous routes to cellulose ester production have been proposed, only a selected few are used industrially. Although specific methodologies vary from manufacturer to manufacturer, two broad categories exist:
1. Homogeneous Acetylation (Solution Acetate Process):
Glacial Acetic Acid Process: Utilizing glacial acetic acid as the solvent, this method allows for controlled acetylation and hydrolysis, achieving desired levels of substitution throughout the polymer chain.
Methylene Chloride Process: Replacing acetic acid with methylene chloride as the solvent provides a similar outcome, with the advantage of faster reaction times.
2. Heterogeneous Acetylation (Fiber Acetate Process):
This method forms cellulose triacetate fibers directly, mimicking the nitration of cellulose. However, it lacks the ability to uniformly hydrolyze the acetyl groups, limiting its versatility compared to the solution processes.
The Glacial Acetic Acid Solution Process:
Figure 1 shows a typical flow diagram for this widely used technique. Briefly, the process involves:
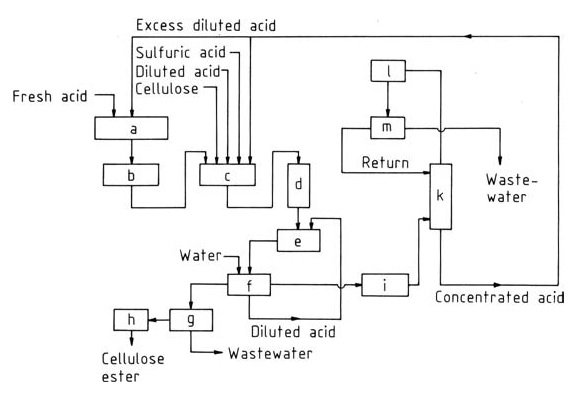
a) Acid reconditioning; b) Acetic anhydride; c) Esterification; d) Hydrolysis; e) Precipitation; f) Washing; g) Centrifuge; h) Drier; i) Evaporator; k) Azeotropic distillation; l) Cooler; m) Decanter
- Pre-treatment: Cellulose is purified and appropriately swollen, typically in acetic acid and sulfuric acid, for efficient acetylation.
- Acetylation: Cellulose reacts with acetic anhydride in the presence of sulfuric acid catalyst, converting hydroxyl groups to ester linkages and forming cellulose triacetate.
- Hydrolysis: To achieve the desired degree of substitution, a controlled hydrolysis step removes some acetyl groups using water and appropriate temperature control.
- Neutralization: The catalyst is neutralized, typically with calcium acetate, to stabilize the final product.
- Filtration and Washing: The cellulose acetate solution is filtered, washed, and concentrated before further processing into films, fibers, or other desired forms.
2.1. Pretreatment of Cellulose
From a purely chemical standpoint, esterifying cellulose hydroxyl groups and then hydrolyzing those esters might seem straightforward. However, the unique architecture of cellulose fibers presents practical challenges that demand specialized processing techniques.
One crucial step is pretreatment, also known as activation. This involves swelling the fibers to facilitate efficient diffusion of acetylation chemicals during the following esterification reaction.
While various swelling agents like water, alcohols, and amines are theoretically possible, acetic acid is the most commonly used in commercial cellulose acetate production. Non-acetic acid options require replacement with acetic acid before acetylation.
Ideally, the cellulose contains 4-7% moisture content. This value depends heavily on the producer’s drying practices and the ambient humidity before use. While inherent moisture aids activation, it causes additional costs by reacting with anhydride during esterification. Conversely, low moisture inhibits activation and slows down the esterification reaction.
Acetic acid-to-cellulose ratios, activation times, and temperatures vary across manufacturers. Often, a small amount of sulfuric acid is added to the activation medium. This further enhances fiber swelling and allows for even catalyst distribution before acetylation.
Additionally, the catalyst induces controlled chain scission in the cellulose by hydrolyzing glycosidic linkages. The extent of chain scission depends on factors like temperature, catalyst uniformity, and water content. Higher pulp moisture retards chain scission by solvating the strong acid catalyst.
2.2. Esterification of Cellulose
The esterification of the hydroxyl groups of cellulose is achieved by two dominant routes: the acetic acid process and the methylene chloride process.
1. Acetic Acid Process
A heterogeneous mixture consisting of glacial acetic acid, acetic anhydride (10–40%), activated cellulose, and a 2-15% sulfuric acid catalyst initiates the reaction. The reaction commences with a rapid exothermic interaction between water within the activated cellulose and a portion of the acetic anhydride.
Unesterified and partially esterified cellulose fibers remain dispersed throughout the reaction medium. Temperature control via vessel cooling and pre-chilled reagents is used to reach 50 °C.
Complete esterification induces fiber dissolution, forming a highly viscous solution. Controlled chain scission, mediated by catalyst levels, temperature, time, and acid-to-anhydride ratio, optimizes solubility in the reaction medium and final product properties.
If the reaction is allowed to proceed to the fully substituted cellulose triacetate via replacement of the remaining sulfate ester groups with acetyl groups, the solution viscosity will increase dramatically until the reaction mass becomes gelled.
After complete fiber dissolution, water or dilute acetic acid quenches the reaction, consuming excess anhydride and preparing for subsequent hydrolysis.
Cooled kneaders with intensive mixing capabilities ensure efficient mixing and catalyst distribution and are important for reaction control. (Figure 2)
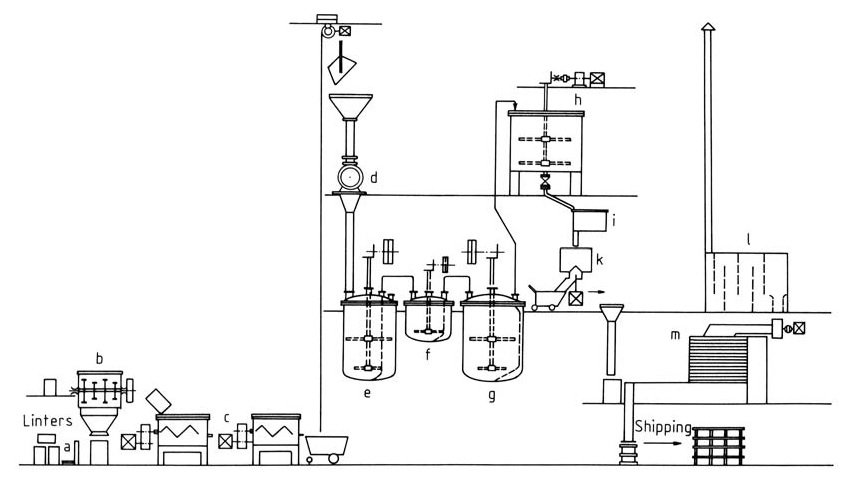
a) Weighing scale; b) Sprinkling vat; c) Kneader; d) Mill; e) Rinsing vat; f) Stabilizing vat; g) Bleaching vat; h) Floater; i) Stock pan; k) Centrifuge; l) Dust chamber; m) Drier
2. The Methylene Chloride Process
Methylene chloride offers several benefits over acetic acid, including lower catalyst requirements, effective heat removal via vaporization (facilitating reaction control), and reduced recycle volume. However, higher esterification temperatures are employed compared to the acetic acid process, while catalyst concentrations decrease to 1% sulfuric acid.
Rotating drums or horizontal containers with stirrers are commonly used due to their suitability for viscous solutions. Corrosion resistance is imperative in this process.
To maintain the fibrous structure during esterification, nonsolvents like carbon tetrachloride, benzene, or toluene are used as the reaction medium. However, uniform hydrolysis is difficult in this heterogeneous system, limiting its application to specific uses like triacetate foils and films.
Perforated drums with rotating capabilities efficiently handle mixing and processing in this process.
2.3. Hydrolysis
The solution esterification process yields a solubilized cellulose triacetate, commercially known as primary acetate, with an approximate acetyl degree of substitution (DS) of 2.9. However, cellulose diacetate (secondary acetate, acetyl DS ~2.5) constitutes the most prevalent commercially available hydrolyzed cellulose acetate product.
Following esterification, water is added and its content adjusted to 5-10%, affecting the final ratio of primary and secondary hydroxyl groups. Typical hydrolysis temperatures vary between 40 and 80°C, primarily dictated by catalyst concentration and temperature. Higher water concentrations can reduce excessive chain scission during hydrolysis.
Hydrolysis progress is monitored by tracking the solubility of the secondary acetate. Upon completion, the sulfuric acid catalyst is neutralized with magnesium, sodium, or calcium acetate. This neutralization also targets the remaining trace sulfate groups bound to the polymer, enhancing stability during isolation and subsequent processing steps.
2.4. Precipitation and Processing
Following hydrolysis, transforming the viscous cellulose acetate solution into a solid form for further processing involves precipitation. Two methods are available:
- Direct Precipitation: Pouring the solution into water (optionally with diluted acetic acid) induces immediate precipitation, resulting in flake-like particles.
- Controlled Precipitation: Gradually adding a diluted acetic acid solution to the stirred solution allows finer control over particle formation, typically yielding a powder-like product.
The chosen method and specific precipitation conditions, including acid concentration, agitation intensity, and temperature, critically influence the final particle morphology. Careful optimization ensures an open pore structure, facilitating efficient removal of residual acids and salts during subsequent water washing.
For the methylene chloride process, solvent must be completely removed via distillation before precipitation. The resulting precipitate is then washed, with the recovered dilute acetic acid recycled back into the production cycle.
Continuous countercurrent washing systems are preferred for thorough purification. High-quality plastic applications may require additional stabilization and bleaching steps. Residual combined sulfate groups can be eliminated by pressure boiling or treatment with dilute mineral acids during stabilization.
Following further rinsing and water removal (e.g., suctioning, centrifugation, pressing, or thrust extraction), the cellulose acetate is carefully dried, ideally in a vacuum shovel drier, to achieve a final moisture content less than 1-3%. An efficient process can achieve a cellulose acetate yield exceeding 95% of the theoretical maximum.
3. Uses of Cellulose Acetate
Examples of cellulose acetate in everyday products include eyeglass frames, cigarette filters, certain textiles in clothing, and even the ink reservoirs in felt-tip pens.
Cellulose acetate is used in diverse commercial sectors, primarily as films, fibers, plastics, and coatings. While cellulose triacetate and diacetate have distinct uses, this section focuses on cellulose diacetate, often simply referred to as cellulose acetate for commercial purposes.
Cellulose Triacetate is used in:
- Film Base: Since the 1950s, cellulose triacetate has served as a clear, smooth film base for photographic emulsions due to its optical isotropy, clarity, toughness, and scratch/moisture resistance.
- Liquid Crystal Displays (LCDs): Recently, cellulose triacetate has found a niche in protecting polarizing films within LCDs for various devices like TVs, monitors, and mobile phones, because of its high moisture vapor transmission rate.
Cellulose Diacetate is used in:
- Films: Due to its wider compatibility with solvents and plasticizers, cellulose diacetate excels in various film applications. Pressure-sensitive tapes, packaging windows, and protective laminates benefit from its transparency, dimensional stability, and tear resistance. Additionally, it can be cast into specific porosities for reverse osmosis membranes in water purification.
- Coatings: Replacing the flammable nitrocellulose, cellulose acetate has served in coatings since WWI. Acetone-based coatings boast fast drying, while other solvents and plasticizers are added for desired rheology and film properties. Applications include lacquers for insulators, glass, paperboard, food packaging, and more.
- Pharmaceuticals: Manufactured using cGMP techniques, cellulose acetate finds use in osmotic drug and sustained-release systems.
- Biodegradability: Under specific conditions, including low acetyl substitution, suitable morphology, and chosen plasticizers, cellulose acetate can biodegrade in certain environments.
Reference
- Cellulose Esters; Ullmann’s Encyclopedia of Industrial Chemistry. – https://onlinelibrary.wiley.com/doi/10.1002/14356007.a05_419.pub2