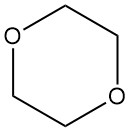
Dioxane, or 1,4-dioxane, is a heterocyclic diether with the formula C4H8O2. It is a flammable, colorless liquid with a faint, sweet odor similar to diethyl ether. It is known by other names such as p-dioxane, diethylene oxide, diethylene dioxide, diethylene ether, 1,4-dioxacyclohexane, dioxyethylene ether, and dioxan.
Dioxane was first produced by A. V. Lourenço in 1863 by reacting ethylene glycol with 1,2-dibromoethane. However, commercial production did not occur until 1929.
Table of Contents
1. Physical Properties of Dioxane
Dioxane is a good solvent with exceptional properties. It readily dissolves a wide range of organic compounds, including aliphatic and aromatic hydrocarbons, ethers, alcohols, ketones, and chlorinated hydrocarbons, especially those with lower molecular weights.
1,4-dioxane is miscible with water in all proportions and also dissolves various inorganic compounds and elements, including iron chlorides, mercuric chloride, mineral acids (hydrochloric, sulfuric, and phosphoric), halogens (bromine, chlorine, and iodine), animal and vegetable oils, paraffin oils, and synthetic and natural resins.
At room temperature, 1,4-dioxane appears as a clear liquid with an ethereal odor. Its chemical stability is comparable to that of other aliphatic ethers. Similar to these ethers, dioxane forms peroxides upon exposure to air.
The most important physical properties of dioxane are listed in Table 1.
Property | Value |
---|---|
Molecular Weight | 88.11 g/mol |
Boiling Point | 101.3 °C |
Freezing Point | 11.8 °C |
Density | 1.0356 g/cm3 |
Latent Heat of Evaporation | 413 kJ/kg |
Heat of Fusion | 141 kJ/kg |
Specific Heat (20 °C) | 1.76 kJ kg-1 K-1 |
Refractive Index | 1.4224 |
Vapor Pressure (20 °C) | 4.13 kPa (41.3 mbar) |
Dielectric Constant (20 °C) | 2.23 |
Surface Tension (20 °C) | 36.9 mN/m |
Critical Temperature | 312 °C |
Critical Pressure | 5.14 MPa |
Flash Point (closed cup) | 11 °C |
Flammable Limits (in air) |
Lower: 2 vol % Upper: 22 vol % |
Heat of Combustion | 27 600 kJ/kg |
Autoignition Temperature | 180 °C |
Dioxane forms azeotropic mixtures with many solvents. Common examples are given in Table 2.
Component | Component bp, °C | Dioxane, wt % | Azeotropic bp, °C |
---|---|---|---|
Formic acid | 100.7 | 57 | 113.35 |
Nitromethane | 100.8 | 43.5 | 100.55 |
Acetic acid | 117.9 | 20.5 – 23 | 119.4 |
Ethanol | 78.5 | 9.3 | 78.1 |
3-Iodo-1-propene | 103 | 44 | 98.5 |
1-Propanol | 97.4 | 45 | 95.3 |
tert-Amyl alcohol | 102.5* | 20 | 100.65 |
Cyclohexane | 80.7 | 24.6 | 79.5 |
Heptane | 98.4 | 44 | 91.85 |
Water | 100 | 82 | 87.8 |
* At 8 kPa |
2. Chemical Reactions of Dioxane
Similar to other ethers, dioxane readily forms peroxides upon exposure to air. These peroxides are often highly explosive and unstable. The primary peroxide formed is 2-hydroperoxy-1,4-dioxane (1), which decomposes to yield dioxanone (2) and water.
Subsequent hydrolysis leads to the formation of 2-(2-hydroxyethoxy)acetic acid (3). Fortunately, the reaction pathway favors the formation of the final product, limiting peroxide concentration.

Despite this, handling dioxane requires the same precautions as with other ethers to prevent peroxide formation. Inert gas sparging, typically with nitrogen, is a recommended practice. Also, peroxides should never be concentrated or distilled to dryness.
Established methods for their removal involve reducing agents like iron(II) or tin(II) chlorides, sodium bisulfite, or adsorbent materials such as activated carbon, alumina, or anion-exchange resins.
1,4-dioxane is able to dissolve and form complexes with various halogens (Cl2, Br2, and I2) and halogen acids. These complexes can moderate the reactivity of the halogens or halogen acids during reactions. As an example, the dioxane dibromide complex can be used for controlled bromination of phenols, aromatic aldehydes/amines, and ketones.
The chlorination of dioxane can yield chlorinated derivatives containing 1 to 8 chlorine atoms. The specific isomer distribution depends on the reaction conditions (temperature, catalysts) employed.
Dioxanes with fewer chlorine atoms are used as precursors for addition compounds, such as ethoxy or ethyl groups, using appropriate reagents like NaOCH2CH3 or CH3CH2MgBr, respectively.
2,3-dichloro-1,4-dioxane is highly reactive, readily condensing with other functional groups like carboxylic acids, alcohols, and glycols to form the corresponding diesters and ethers.
While the dioxane ring is stable, strong acids under elevated temperature and pressure can cleave it. Under such conditions, reactions with acetic anhydride yield ethylene glycol diacetate and diethylene glycol diacetate.

Dioxane can be decomposed by hydrogen peroxide at 25 °C using FeSO4 as a catalyst.
Lewis acid-catalyzed reactions of 1,4-dioxane with benzoyl chloride or acetyl chloride produce the 2-chloroethyl esters of benzoic acid and acetic acid, respectively.
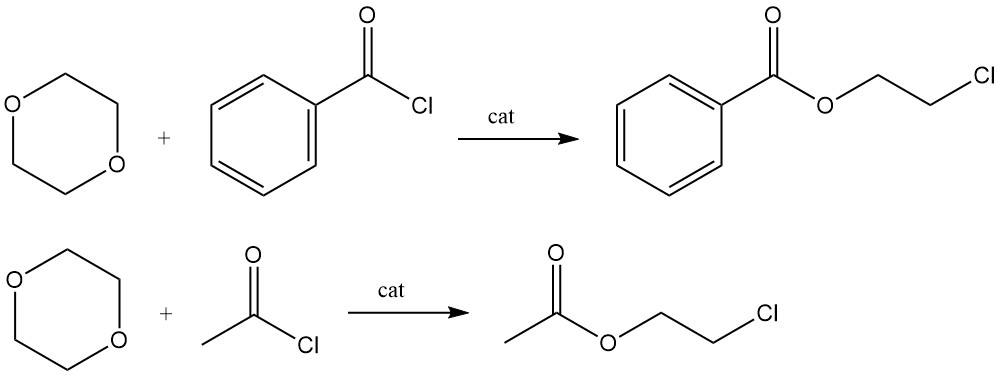
Using benzoyl peroxide as a catalyst, dioxane reacts with α-olefins at a temperature of 80–140 °C for 5–10 h to produce a variety of polymers used as lubricants.
Similar reactions can occur with tetrafluoroethylene, where peroxide-catalyzed reactions at high temperatures and pressure yield analogous polymers.
Numerous alternative catalysts have been reported for these polymerizations, including peroxides (organic and inorganic), bases (borax, disodium phosphate, hydrazine), and Lewis acids (zinc chloride, phosphoric acid).
Other than halogens, 1,4-dioxane forms complexes with various functional groups, such as AlBr3, AlCl3, AuCl3, BBr3, BCl3, BF3, CuCl2, CoCl2, FeCl3, HgCl2, LiBr, LiCl, LiI, ZnCl2, PtCl4, HCl, H2SO4, H3PO4, SO2, SO3, and trinitrophenol.
These complexes are used as catalysts, moderating agents in reactions like bromination or sulfonation, or as reaction media for anhydrous acid systems.
3. Production of Dioxane
Dioxane is primarily produced by a closed-loop process involving the dehydration and subsequent ring closure of diethylene glycol. Concentrated sulfuric acid (around 5%) is used as the catalyst for this reaction. However, alternative catalysts like phosphoric acid, p-toluenesulfonic acid, and strongly acidic ion-exchange resins can also be employed.
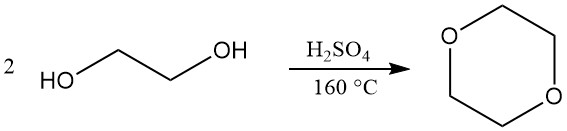
The operating conditions for this process can vary. Reaction temperatures typically range from 130 to 200 °C, while pressure can vary from slightly above atmospheric pressure to a partial vacuum (25–110 kPa). A reaction temperature of 160 °C is often considered optimal.
The reaction setup allows for continuous production, with the formed dioxane vaporized directly from the reaction vessel. These vapors are then passed through an acid trap and a series of distillation columns to remove water and purify the final product. Yields of approximately 90% are achievable.
It’s important to note that this process generates unwanted byproducts such as 2-methyl-1,3-dioxolane, acetaldehyde, crotonaldehyde, and polyglycol.
While the dehydration of diethylene glycol is the dominant commercial route, other methods exist for synthesizing 1,4-dioxane. These less common methods include:
- Dehydrohalogenation of 2-chloro-2′-hydroxyethyl ether
- Reaction of ethylene glycol with 1,2-dibromoethane
- Dimerization of ethylene oxide using catalysts like NaHSO4, SiF4, or BF3, or by high-temperature reactions with acidic cation-exchange resins
4. Uses of Dioxane
The most important industrial application of 1,4-dioxane is the stabilization of 1,1,1-trichloroethane against reaction with aluminum. An aluminum oxide film forms on the metal surface, preventing direct contact with 1,1,1-trichloroethane.
However, when this protective layer is disturbed, 1,1,1-trichloroethane reacts with the exposed aluminum, extracting chlorine and forming aluminum chloride, which then promotes the dehydrohalogenation of 1,1,1-trichloroethane to produce hydrochloric acid and vinylidene chloride.
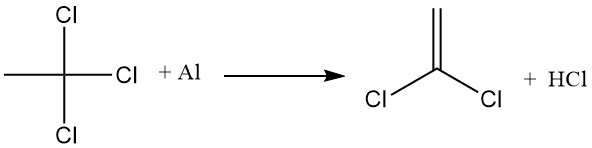
1,4-dioxane inhibits these reactions by forming an insoluble complex between dioxane and aluminum chloride. This complex deactivates the AlCl3 catalyst and seals any openings in the aluminum oxide film, preventing direct contact between the metal and 1,1,1-trichloroethane.
Dioxane is employed as a wetting and dispersing agent in textile processing, dye baths, staining, and printing compositions. Additionally, it aids in the preparation of histological slides.
Dioxane is used in other sectors, including:
- Solvents: It is used as a solvent in formulating inks, coatings, adhesives, scintillation counters, and for extracting animal and vegetable oils.
- Chemical Intermediate: products derived from dioxane are valuable as insecticides, herbicides, plasticizers, and monomers.
- Catalysis and Reagents: Oxonium complexes formed by dioxane with salts, mineral acids, halogens, and sulfur trioxide function as catalysts and reagents in anhydrous acid reactions, brominations, and sulfonations.
- Laboratory Applications: In laboratories, 1,4-dioxane is used as a cryoscopic solvent for determining molecular masses, and its stability makes it a suitable reaction medium for various chemical transformations.
- Traditionally, dioxane was used in polymer manufacturing and as a solvent for natural and synthetic resins, including cellulose derivatives, polyvinyl acetal resins, and acrylonitrile-methyl vinyl pyridine copolymers.
5. Toxicology of Dioxane
Dioxane has a relatively low acute toxicity. Oral and dermal LD50 values in rats and rabbits, respectively, are 5170 mg/kg and 7600 mg/kg. Inhalation exposure for 4 hours at 14,260 ppm (51,880 mg/m³) is lethal to 50% of the exposed rat population (LC50).
Occupational Exposure Limits:
- American Conference of Governmental Industrial Hygienists (ACGIH) Threshold Limit Value—Time-Weighted Average (TLV-TWA): 25 ppm (90 mg/m³) with a “skin” notation.
- US Occupational Safety and Health Administration (OSHA): 100 ppm (360 mg/m³)
- Deutsche Forschungsgemeinschaft (MAK): >50 ppm (180 mg/m³) with a IIIB notation (suspected carcinogen)
Exposure Control Measures
Due to the potential for skin and eye irritation and respiratory system damage at high concentrations, workplaces using 1,4-dioxane should implement control measures to minimize worker exposure. These include engineering controls to minimize airborne vapor levels and the use of personal protective equipment such as gloves, eye protection, and respirators, when necessary.
Carcinogenicity
Early animal studies using high oral doses of dioxane (7–18,000 mg/kg) resulted in nasal and liver tumors. However, subsequent studies at lower doses (1000 mg/kg and below) showed no tumor formation, suggesting a possible threshold effect. Additionally, mutagenicity tests did not reveal any direct interaction of dioxane with DNA.
These findings suggest that tumors in rodents may arise from repeated tissue damage rather than direct genotoxicity.
Metabolism and Elimination
Studies using radiolabeled dioxane indicate that low doses are rapidly converted to and eliminated in urine as 2-hydroxyethoxyacetic acid. This conversion process becomes less efficient at high doses, resulting in slower elimination.
Human Exposures and Carcinogenicity Risk Assessment
Human exposure incidents at estimated concentrations of 470 ppm of dioxane have resulted in fatalities. However, epidemiological studies of workers exposed to levels up to 51 ppm haven’t shown any adverse health effects.
The potential carcinogenicity of 1,4-dioxane has prompted various international regulatory bodies to establish guidelines for its presence in drinking water and the environment.
The International Agency for Research on Cancer (IARC) classifies 1,4-dioxane as a Group 2B carcinogen (probably human carcinogen) based on animal studies showing increased incidence of nasal cavity, liver, and gallbladder carcinomas in rats, mice, and guinea pigs, respectively.
Dioxane in Drinking Water Guidelines
The World Health Organization (WHO) recommends a guideline value of 50 μg/L for 1,4-dioxane in drinking water.
The U.S. Environmental Protection Agency (EPA) and the National Center for Environmental Assessment proposed a health-based advisory level of 3 μg/L in drinking water.
The EPA’s Integrated Risk Information System suggests a potential cancer risk of 1 in 1,000,000 at a lifetime exposure of 0.35 μg/L. Consequently, the Unregulated Contaminant Monitoring Rule (UCMR3) sets a minimum reporting level of 0.07 μg/L.
The German Federal Environmental Agency recommends a precautionary guideline limit of 0.1 μg/L for non-genotoxic carcinogens like 1,4-dioxane in drinking water.
Japan established an environmental standard of 50 μg/L for 1,4-dioxane.
In the United States, several individual states have set their own advisory levels for 1,4-dioxane in drinking water, reflecting a patchwork of regulations across the country.
References
- Dioxane; Ullmann’s Encyclopedia of Industrial Chemistry. – https://onlinelibrary.wiley.com/doi/10.1002/14356007.a08_545.pub2
- 1,4-Dioxane; https://onlinelibrary.wiley.com/doi/10.1002/9781119407621.ch4