The production of amines involves different methods, each suited to specific raw materials and desired products. Aliphatic amines are produced from alcohols, carbonyl compounds, nitriles, alkyl halides, nitro compounds and olefins.
Table of Contents
1. Production of Amines from Alcohols
The most prevalent technique for synthesizing lower alkylamines involves the reaction between a suitable alcohol and ammonia using an appropriate catalyst. This reaction yields a mixture of primary, secondary, and tertiary amines due to the ability of the initially formed primary amine to react further with one or two alcohol molecules.
While the conversion of alcohol to primary amine exhibits thermoneutral characteristics, the formation of secondary and tertiary amines is exothermic, favoring thermodynamics. Additionally, the primary amine exhibits higher reactivity than ammonia due to its greater nucleophilic nature.
The distribution of products can be partially controlled by adjusting reaction conditions such as temperature, excess ammonia, and residence time. As the obtained mixture of amines often fails to meet market requirements, non-marketable amines can be recycled, thereby increasing the yield of the desired amine to over 90%.
RCH2OH + NH3 ↔ RCH2NH2 + H2O
RCH2NH2 + RCH2OH ↔ (RCH2)2NH + H2O
(RCH2)2NH + RCH2OH ↔ (RCH2)3N + H2O
Previously, this reaction was conducted using pure dehydration catalysts like alumina, silica, titania, thorium oxide, tungsten oxide, chromium oxides, phosphates, or various mixed oxides (e.g., silica-alumina, clays, zeolites) at temperatures up to 500 °C. However, the use of these catalysts is now limited to methylamine production.
For the conversion of alcohols containing two or more carbon atoms, catalysts with hydrogenating and dehydrogenating properties have gained importance. Nickel, cobalt, copper, iron, platinum, or palladium-based catalysts are primarily employed, with noble metal catalysts exhibiting a tendency to cause C-C or C-N bond cleavage, leading to lower selectivities. Promoters like Ag, Zn, In, Mn, Mo, and alkali metals are added to enhance catalyst performance.
Typically, the active components are supported on carriers such as Al2O3, SiO2, or ZrO2. Zeolites have been proposed as carriers to improve selectivity towards primary amines. In this continuous process, the alcohol, ammonia, and hydrogen are passed over the catalyst in a fixed-bed reactor. The reaction occurs at pressures of about 0.5-25 MPa and temperatures of approximately 100-250 °C, depending on the catalyst and the choice between liquid-phase and gas-phase processes.
The initial and rate-determining step is the dehydrogenation of the alcohol to form the carbonyl compound. Subsequent addition of ammonia leads to water loss and the formation of an imine or an enamine, which is then hydrogenated to yield the final amine.
Side reactions include amine disproportionation, particularly at higher reaction temperatures, and to a lesser extent, aldol condensation of intermediate aldehydes, formation of Schiff base between these aldehydes and the amine product, and nitrile formation at high temperatures and low hydrogen pressures.
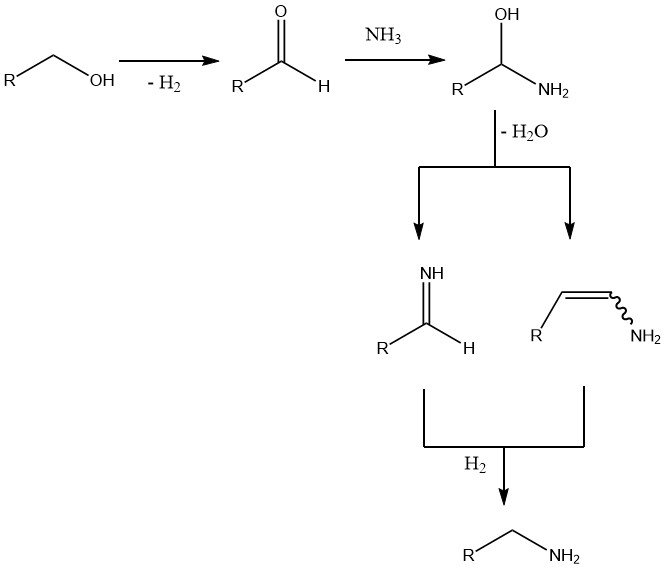
An excess of two to eight times the amount of ammonia is utilized to shift the equilibrium towards the primary amines. While hydrogen is not directly involved in the reaction, its absence results in the formation of imines, enamines, and even nitriles.
Furthermore, hydrogen helps maintain catalyst activity by removing carbonaceous deposits and metal carbides or nitrides, as well as preventing disproportionation of the amine products.
The amination of diols and polyols follows similar principles to monoalcohols, although the occurrence of side reactions such as cyclizations complicates the process.
The same methodology can be employed to alkylate primary or secondary amines instead of ammonia. When an amine and an alcohol with different aliphatic substituents react, a mixed aliphatic amine is produced. For instance, N,N-dimethylethylamine can be synthesized from dimethylamine and ethanol.
To prevent transalkylation at the nitrogen atom during such reactions, copper catalysts are recommended. Copper chromite, in particular, is an effective catalyst for long-chain tertiary amines like dimethyldodecylamine.
2. Production of Amines from Carbonyl Compounds
If it is more cost-effective, aldehydes and ketones are preferred over alcohols for amine synthesis. However, this is generally limited to lower aldehydes obtained from oxo synthesis and acetone produced as a byproduct in phenol production (Hock synthesis).
The reaction between a carbonyl compound and ammonia or an amine occurs in two steps. Initially, an imine or Schiff base is formed, which is then hydrogenated with hydrogen in a second step to yield the amine. The typical procedure is similar to alcohol conversion, where the reaction mixture containing the carbonyl compound, ammonia, and hydrogen is passed over a fixed-bed catalyst.
In some cases, it may be beneficial to carry out the reaction in two stages. The carbonyl compound and ammonia or amine react first, the resulting water is removed, and then the hydrogenation step is performed.

The key difference between aminating an aldehyde or ketone and an alcohol is that hydrogen acts as a reactant and is consumed in a stoichiometric amount in the former case.
Due to the considerably higher heat of reaction in this process (e.g., 60.4 kJ/mol for acetone compared to 7.1 kJ/mol for isopropyl alcohol), a different reactor design is required.
Typically, the process is conducted in the vapor phase at temperatures of 100-160 °C and atmospheric or slightly above atmospheric pressure. High-pressure processes can also be employed if the heat of reaction is effectively removed, such as through a high recycle rate or using a multitubular reactor. Lower reaction temperatures compared to alcohol reactions generally allow for higher selectivities in principle.
In general, the same catalysts used for the hydrogenative amination of alcohols can be utilized. Control of product distribution by adjusting the excess ammonia and distillation-based workup are also similar. Detailed reviews discussing industrial and preparative possibilities for synthesizing amines from carbonyl compounds are available.
While alternative reducing agents can be used instead of hydrogen, their industrial significance is limited due to challenges in handling and their cost (e.g., selenophenol or NaBH4).
The Leuckart-Wallach reaction, which employs ammonium formate to aminate the carbonyl compound with the generation of CO2 as a by-product, has found some application.
3. Production of Amines from Nitriles
In many cases, nitriles are more cost-effective to obtain than their corresponding alcohols or carbonyl compounds. There are several methods for synthesizing nitriles, including ammoxidation, addition of an amine or alcohol to acrylonitrile, or conversion from the corresponding carboxylic acid.
To convert nitriles into the corresponding amines, catalytic hydrogenation is preferred. Noble metal catalysts such as palladium, platinum, and rhodium, as well as nickel, cobalt, and increasingly iron catalysts, are commonly employed.
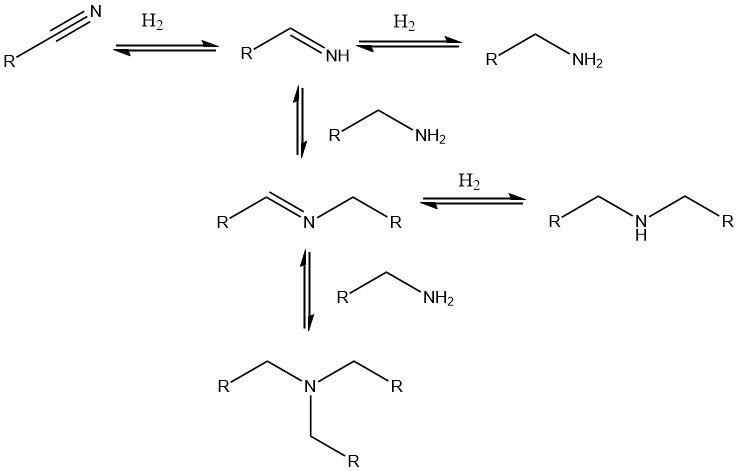
Supported noble metal catalysts allow for milder reaction conditions (20-100 °C, 0.1-0.5 MPa), while nickel or cobalt catalysts require higher pressures of up to 25 MPa and temperatures up to 180 °C. When di- or polynitriles are hydrogenated to di- or polyamines, a base like NaOH is often added to the catalyst.
There are different variations of the process, but the most commonly used ones are the batch process with a suspended catalyst and the continuous process with a fixed-bed catalyst.
Side reactions leading to the formation of secondary amines are inevitable, but they can be suppressed by adding ammonia, sodium hydroxide solution, or an acid.
Alternatively, process conditions can be adjusted to prioritize the formation of secondary or tertiary amines. This approach is useful, for instance, in the synthesis of di- and triethylamine from acetonitrile or dibutylamine from butyronitrile.
The hydrogenation of acrylonitrile is a special case. While saturated amines are typically the sole products of this reaction, using copper chromite as a catalyst instead of noble metals, nickel, or cobalt leads to the formation of allylamine and diallylamine.
Many significant nitrile hydrogenations to produce the corresponding amines are based on previous addition reactions involving acrylonitrile. Alcohols can add to acrylonitrile only in the presence of a basic catalyst like NaOH, KOH, or quaternary ammonium hydroxides.
Since amines themselves are sufficiently basic, the addition occurs readily. In some cases, higher temperatures may be required for cyanoethylation, and stabilizers have been described to prevent the polymerization of acrylonitrile.
4. Synthesis of Amines from Alkyl Halides
When an alkyl halide reacts with ammonia or an amine, an alkylammonium halide is formed, which can be converted to the amine by treatment with a strong alkaline solution. Although this method is commonly used in preparative chemistry, it has limited industrial significance, mainly employed for the production of ethylenediamine, polyamines, and a few specialized amines like allylamine and certain pharmaceuticals with low production volumes.
The reasons for its limited use on an industrial scale are the high cost of starting materials, challenges related to corrosion and product quality during halide processing, and the need for disposal of the resulting salt byproduct.
5. Synthesis of Amines from Nitro Compounds
While the reduction of nitro compounds is a vital method for synthesizing aromatic amines, it has not gained significant importance in the production of aliphatic amines. Due to limited availability of suitable nitroalkanes, this method is only employed in specific cases, such as the production of 2-amino-2-methyl-1-propanol used in the formulation of buffer solutions, latex coatings, metalworking fluids, and cosmetics.
The reduction process involves the formation of a nitroso or hydroxylamine intermediate, and in some cases, can occur at room temperature, yielding amine products with 90% or higher yields. Hydrogenation catalysts such as platinum, palladium, rhodium, nickel, or copper are utilized.
The reaction is highly exothermic (approximately 500 kJ/mol per nitro group), necessitating efficient heat removal for process control. Nitroparaffins pose greater challenges for hydrogenation compared to their aromatic counterparts.
Functionalization of alkenes using NO or NO2 has been reported, proceeding through a radical mechanism and resulting in a mixture of products including nitroalkenes. However, the reduction of nitroalkenes to amines has not found industrial application thus far. Similarly, primary amines have been obtained from paraffins by nitration with HNO3 or liquid NO2, followed by hydrogenation of the resulting mixture of nitroparaffins and ketones in the presence of ammonia.
6. Production of Amines from Olefins
Amines that have a tertiary alkyl group next to the nitrogen atom, such as tert-butylamine, are challenging to synthesize using conventional methods. However, these compounds can be readily prepared by adding hydrogen cyanide to an alkene, such as 2-methylpropene, in an acidic medium.

This reaction, known as the Ritter reaction, is typically carried out at temperatures of 30-60 °C. The resulting formamide intermediate is then hydrolyzed by heating the reaction mixture to approximately 100 °C.
To isolate the amine, the acidic mixture is neutralized, resulting in the formation of sodium formate and the salt of the original acid (e.g., sodium sulfate).
Due to the difficulties associated with handling hydrocyanic acid, which is corrosive and poses safety concerns, one carbon atom is lost as formate during the reaction, and a significant amount of salt (approximately 3.3 kg/kg tert-butylamine) must be disposed of in deep wells. As a result, this process is limited to the synthesis of amines that cannot be obtained using other methods.
Although the direct addition of ammonia or an amine to an olefinic double bond is thermodynamically favorable, it is limited to a few specific cases involving activated amines or compounds with activated double bonds. An example is the addition of an amine to acrylonitrile.
However, it is possible to achieve the addition of ammonia to non-activated alkenes under pressure using catalysts. Acidic zeolites are particularly effective catalysts for this reaction. The first successful demonstration was the formation of ethylamines from ethylene and ammonia using H-mordenite.
Although the conversion in the direct amination of alkenes is typically low due to kinetic and thermodynamic limitations, the selectivity for monoalkylamines can be excellent with the appropriate choice of zeolite and alkene. Small-pore zeolites are well-suited for the production of ethylamines, while medium-pore zeolites can be used for tert-butylamine synthesis. Certain large-pore zeolites have shown improvements over medium-pore zeolites.
High selectivities (>95%) with acceptable conversions (10-15%) have been achieved in the amination of isobutene over RE-Y-zeolites and Al-, Fe-, or B-substituted pentasil-type zeolites at temperatures of 250-300 °C and pressures around 30 MPa. These zeolites exhibit excellent long-term stability compared to X- and Y-zeolites.
Homogeneously catalyzed amination of alkenes is limited to specific cases. Although not highly selective, the hydroformylation of alkenes in the presence of ammonia or amines shows potential for future applications. However, this method currently faces challenges, as significant amounts of alcohols and dimerization products are formed in addition to the desired amines derived from n-aldehyde and isoaldehyde.
7. Other Processes
The hydrogenation of aromatic amines to cycloalkylamines is primarily limited to the production of cyclohexylamines on an industrial scale. Other cycloalkylamines are not commonly synthesized through this method at an industrial level.
There has been a description of a process for synthesizing amines from carbon monoxide, hydrogen, and ammonia or amines using an activated iron catalyst based on the Fischer-Tropsch-type reaction. However, this method is not widely employed on an industrial scale.
The reduction of amides and other synthetic routes mentioned in the literature, while existing, are mainly laboratory methods suitable for small-scale preparations. These methods are rarely used at an industrial level, with only isolated cases where they are implemented.
Reference
- Amines, Aliphatic; Ullmann’s Encyclopedia of Industrial Chemistry. – https://onlinelibrary.wiley.com/doi/10.1002/14356007.a02_001.pub2