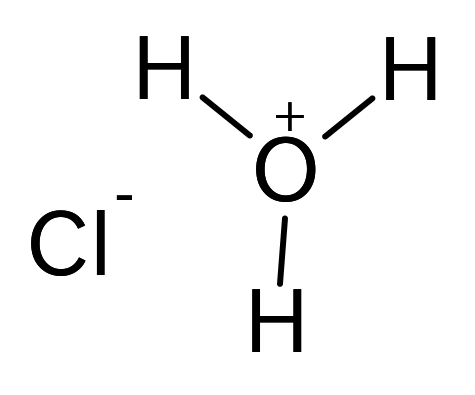
Hydrochloric acid, known by its alternative name muriatic acid, is a solution of strong inorganic acid with the chemical formula HCl. It is a colorless liquid with a pungent odor that is very corrosive to skin, eyes and metals.
History of Discovery
During the 15th century, a German alchemist named VALENTIN applied heat to a substance called green vitriol (FeSO4·7 H2O) together with common salt. As a result, he obtained a substance referred to as spirit of salt.
In the 17th century, GLAUBER synthesized hydrochloric acid by combining common salt with sulfuric acid. It was in 1790 when DAVY established the composition of hydrogen chloride through the synthesis of hydrogen and chlorine.
During the same year, LEBLANC discovered a process, named after him, for soda production. This process involved reacting common salt with sulfuric acid, resulting in the release of hydrogen chloride. Initially considered an unwanted byproduct, it was released into the atmosphere in significant quantities.
However, in 1863, the Alkali Act compelled English soda producers to dissolve the hydrogen chloride in water, leading to the widespread industrial use of the produced acid. Any excess hydrogen chloride that could not be utilized as hydrochloric acid was oxidized to chlorine.
The industrial synthesis of hydrogen chloride emerged alongside the development of the chlor-alkali electrolytic process in the early 20th century. This method, in addition to the one based on the reaction between chlorides and sulfuric acid or sodium hydrogen sulfate, yielded a purer product.
These processes are gradually decreasing in significance due to the substantial quantities of hydrogen chloride generated as byproducts in chlorination processes like the production of vinyl chloride from ethylene. Furthermore, hydrochloric acid can be recovered from gases produced during the incineration of chlorine-containing waste, which aligns with the growing focus on environmental protection.
Table of Contents
1. Physical Properties of hydrochloric acid
Hydrogen chloride (HCl) exists in standard conditions as a transparent gas characterized by its strong smell and highly corrosive impact on the respiratory system’s mucous membranes.
Some physical properties of hydrogen chloride are presented in the following table:
Property | Value |
---|---|
Molar Mass | 36.461 g/mol |
Melting Point (mp) | -114.22 °C |
Boiling Point (bp) | -85.05 °C |
Vapor Density | 1.639 g/L |
Liquid Density | 1.18 g/mL |
Critical Temperature | 51 °C |
Critical Pressure | 81.6 bar |
2. Chemical Properties of hydrochloric acid
Hydrogen chloride exhibits thermal stability up to approximately 1500 °C, beyond which it undergoes significant dissociation. In its completely dry form, hydrogen chloride demonstrates limited reactivity, showing negligible attack on mild steel.
Furthermore, reactions involving anhydrous hydrogen chloride typically necessitate the presence of catalysts. Conversely, when hydrogen chloride is dissolved in a polar solvent, such as water, it transforms into a potent acid and consequently becomes a highly reactive reagent.
The solubility of hydrogen chloride in water is substantial, accompanied by the release of a significant amount of heat. This results in the formation of hydrochloric acid, which possesses a formidable and exceedingly aggressive nature.
It exhibits the ability to corrode most commercially available metals and alloys to varying degrees. Consequently, metals necessitate protection through the utilization of suitable nonmetallic linings or nonmetallic construction materials.
Under precisely defined conditions, tantalum or highly resistant nickel alloys like Hastelloy B can be employed. However, less noble metals dissolve, leading to the liberation of hydrogen gas.
3. Production of Hydrochloric Acid (HCl)
The presence of free hydrogen chloride in nature is limited, occurring only in low concentrations. It has been detected in the stratosphere at levels of approximately 10–11 volume percent.
On the other hand, inorganic chlorides have a wide distribution, with chlorine ranking as the eleventh most abundant chemical element. Alkali-metal and alkaline-earth chlorides, particularly rock salt, serve as the primary raw materials for various methods of hydrogen chloride or hydrochloric acid production.
These methods include processes involving intermediate substances like chlorine or chlorinated organic compounds.
In natural occurrences, hydrogen chloride is released during volcanic eruptions or other releases of subterranean gases. The quantity of hydrogen chloride produced can be comparable to that of sulfur dioxide.
Hydrochloric acid is naturally present in the gastric juice of mammals, with a concentration of approximately 0.1 mol/L in the human stomach.
3.1. Methods of Preparation of Hydrochloric Acid (HCl)
Hydrogen chloride (HCl) is prepared by several reactions:
1. Synthesis from elements:
The combination of hydrogen gas and chlorine gas leads to the formation of hydrogen chloride gas:
H2 + Cl2 → 2 HCl
2. Reaction of metallic chlorides with sulfuric acid:
Metallic chlorides, especially sodium chloride, can react with sulfuric acid or hydrogen sulfate to produce hydrogen chloride:
Metallic chloride + H2SO4 → HCl + Metal sulfate
3. Byproducts of chlorination:
During the production of various chlorinated organic compounds like dichloromethane, trichloroethylene, perchloroethylene, or vinyl chloride, hydrogen chloride is formed as a byproduct through a series of reactions:
C2H4 + Cl2 → C2H4Cl2
C2H4Cl2 → C2H3Cl + HCl
4. Thermal decomposition of hydrated heavy-metal chlorides:
Hydrogen chloride can be derived from the thermal decomposition of hydrated heavy-metal chlorides present in spent pickle liquor used for metal treatment.
5. Incineration of chlorinated organic waste:
When chlorinated organic waste undergoes incineration, hydrogen chloride is produced as a result:
C4H6Cl2 + 5 O2 → 4 CO2 + 2 H2O + 2 HCl
The primary source of hydrogen chloride nowadays is the byproduct obtained from chlorination processes. The purification level required depends on the intended application. Currently, the recovery of hydrogen chloride from waste materials is increasing.
Additionally, hydrogen chloride can be formed in undesired reactions like the hydrolysis of Friedel-Crafts catalysts:
AlCl3 + 3 H2O → Al(OH)3 + 3 HCl
3.2. Industrial Production of hydrochloric acid (HCl)
3.2.1. From Hydrogen and Chlorine
The most straightforward method for producing hydrogen chloride is its direct synthesis from its elemental components, resulting in a highly pure product. This reaction is highly exothermic, with a standard enthalpy of reaction (ΔH) of -184 kJ.
It is important to note that mixtures of hydrogen and chlorine can be extremely explosive across a wide range of compositions. Light can serve as an initiator for an explosion. Therefore, precautions must be taken to prevent the formation of reactive mixtures of chlorine and hydrogen in industrial processes.
In industrial production, a burner is employed, where chlorine and hydrogen are separately fed through concentric tubes into the combustion chamber. Once ignited, chlorine burns in the presence of hydrogen, generating a hot and quiet flame at temperatures exceeding 2000 °C.
Silica has demonstrated effectiveness as a suitable construction material for the burner. Cast iron or steel, sometimes water-cooled, or graphite have also been utilized.
Typically, the burner is positioned at the bottom of a cylindrical combustion chamber, with the flame directed vertically upwards. The combustion chamber itself is made of steel and lined with refractory brick. A cooling section, appropriately sized and shaped, is connected to the combustion chamber.
If the reactants are highly moist or contaminated, corrosion-resistant construction materials such as silica or graphite must be employed.
Existing manufacturing plants are predominantly constructed using carbon steel, and the hydrogen chloride produced is water-cooled only to a temperature where aqueous hydrochloric acid cannot condense.
The primary contaminants are moisture in hydrogen and oxygen in chlorine, although oxygen compounds like dichlorine oxide (Cl2O) or carbon dioxide can also pose issues by forming water.
The mixing ratio of the raw materials is adjusted based on the intended use of the produced hydrogen chloride. A slight excess of hydrogen or chlorine may be employed.
Working with precisely equimolar mixtures is not recommended since the reaction is not completely quantitative, and it becomes more challenging to prevent explosive mixtures.
Deviations in control can lead to intermittent shifts between excess hydrogen and excess chlorine, resulting in the occasional appearance of explosive mixtures.
Typically, hydrogen in hydrogen chloride presents fewer challenges than chlorine, so a slight excess of 1-2% hydrogen is commonly used. Depending on the purity of the raw materials, it is possible to obtain hydrogen chloride completely free from chlorine, with excess hydrogen being the only impurity.
This highly pure hydrogen chloride can be utilized to produce exceptionally pure hydrochloric acid or can be liquefied or supplied directly to users as a dry gas through steel pipelines.
Using steel for synthesis furnaces and coolers is an attractive option. This enables operation under elevated pressure, which is determined by the pressure of chlorine and hydrogen, as well as the temperature and pressure in the pipeline.
It is crucial to maintain a pressure low enough or a temperature high enough to prevent the condensation of aqueous hydrochloric acid. The steel furnaces operated at H€uls, for instance, can each produce 30-40 tons per day of hydrogen chloride.
The generated hydrogen chloride gas has a pressure of up to 0.7 MPa and a water content of less than 50 ppm (by volume), necessitating the use of steel pipes for transportation from the plant.
Chemically related processes include the reaction of chlorine with carbon (in the form of coke) and water vapor:
C + 2 H2O + 2 Cl2 → 4 HCl + CO2
Another related process involves the reaction of chlorine with sulfur dioxide and water:
SO2 + 2 H2O + Cl2 → 2 HCl + H2SO4
3.2.2. Reaction of Metal Chlorides with Sulfuric Acid
The sulfate process is characterized by high energy consumption. Consequently, its significance has gradually diminished compared to other processes, particularly organic chlorination, which generates significant quantities of hydrogen chloride as a byproduct.
Furthermore, the argument that sulfate-hydrochloric acid yields a product of higher purity is no longer valid. Byproduct acid can now be adequately purified to meet the requirements for high-quality standards. Therefore, only the most important sulfate-hydrochloric acid process will be discussed here.
The reaction between common salt and concentrated sulfuric acid occurs at relatively low temperatures (150-300 °C), resulting in the formation of hydrogen chloride and sodium hydrogen sulfate.
The latter reacts with excess sodium chloride at a minimum temperature of 550-600 °C, producing neutral sodium sulfate. Although using sodium hydrogen sulfate as a starting material is technically feasible, it has been discontinued due to the uneconomical nature of the process caused by the high temperatures (600-800 °C) and energy consumption involved.
Currently, sulfuric acid is the exclusive starting material. In the Mannheim process, sodium sulfate is produced, while in the Berlin hydrochloric acid process, sodium hydrogen sulfate is utilized.
In the Mannheim process, externally fired muffle furnaces lined with bricks are employed. These furnaces include a stirring mechanism with a scraping action to prevent the formation of lumps in the pasty mass and to remove the produced sodium sulfate.
Hydrogen chloride is discharged through the side of the muffle and propelled by a fan to the subsequent stage, with the furnace maintained at slightly reduced pressure.
The exhaust gas mixture from the furnace contains up to 85% hydrogen chloride, along with air, sulfuric acid mist, and fine salt particles. Typically, the gas undergoes multiple purification stages, including filtration through coke and activated carbon, wet scrubbing, or chemical reactions.
The Berlin hydrochloric acid process yields hydrogen chloride gas of significantly higher purity than the Mannheim process. The reaction between common salt and sulfuric acid occurs in molten sodium hydrogen sulfate at around 300 °C within cast-iron retorts.
Alternatively, potassium chloride can be used, resulting in the production of hydrogen chloride and potassium hydrogen sulfate. This process retains some commercial significance due to the primary products being the potassium salts formed (i.e., KHSO4 and K2S2O7), with hydrogen chloride considered a byproduct.
Gas from the retorts is more concentrated due to the improved leak-proof nature of the equipment, resulting in lower levels of sulfuric acid mist as a consequence of the reduced working temperature.
3.2.3. Recovery as a Chlorination Byproduct
The primary source of hydrogen chloride and hydrochloric acid is the byproduct of chlorination processes.The cracking of 1,2-dichloroethane to produce vinyl chloride stands out as the major contributor to hydrogen chloride production.
The quantities of hydrogen chloride generated and consumed can be significant, with many facilities producing around 10,000 m3/h (16 t/h or 140,000 t/a) or more.
Consequently, hydrogen chloride is often directly supplied to chemical plants that utilize it as a raw material. Storage capacities for hydrogen chloride are typically relatively small. Therefore, it is crucial to maintain a balanced rate of production and consumption.
In the event of production issues or market fluctuations affecting one plant or product, the other plant can continue operating only if there is reserve production capacity (which is usually not fully utilized) in place.
Buffer storage for liquid hydrogen chloride is employed to address short-term imbalances between production and consumption.
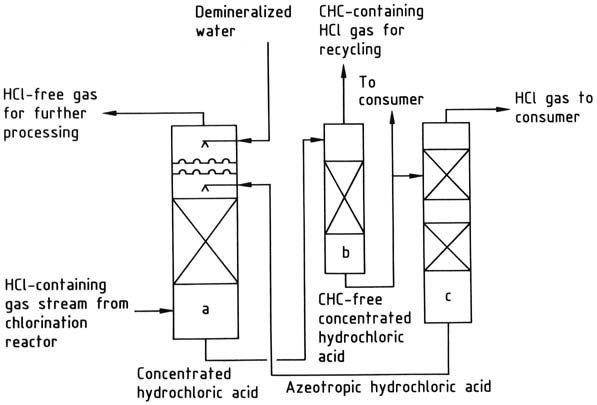
a) Hydrogen chloride absorption column; b) Chlorinated hydrocarbon stripping column; c) Hydrogen chloride desorption column
Hydrogen chloride produced through organic chlorination can undergo various treatment methods:
1. Simple condensation of chlorinated hydrocarbons: This approach is used when the presence of residual chlorinated hydrocarbons or other byproducts from the chlorination process does not pose any issues for the hydrogen chloride, which is supplied as a gas.
The level of purification achieved depends on the condensation pressure and temperature. For instance, in the chlorination of chloromethane to dichloromethane, the resulting hydrogen chloride is solely used to convert methanol to chloromethane, which is subsequently utilized to produce dichloromethane.
2. Isolation and purification of hydrogen chloride by distillation: Fractional distillation of the liquefied gas can be employed when a purer product is required. For example, the gaseous products derived from the cracking of 1,2-dichloroethane are liquefied and then separated through distillation into vinyl chloride and hydrogen chloride.
The liquefaction of these gases at extremely low temperatures is economically impractical, so condensation and distillation are carried out under elevated pressure. An advantage of this method is that hydrogen chloride gas is produced at a sufficiently high pressure (typically 1-2 MPa) for subsequent processing, eliminating the need for additional mechanical compression.
3. Aqueous absorption of hydrogen chloride: This method results in the production of hydrochloric acid as an intermediate product but presents challenges related to construction materials.
However, the introduction of fluoropolymers has mitigated these problems to a large extent. Nevertheless, many installations have replaced aqueous treatment with non-aqueous methods, such as fractional distillation.
Hydrogen chloride can be absorbed using water or a 20 wt % hydrochloric acid solution. Water is suitable when the primary goal is to produce 30-35 wt % hydrochloric acid as the final product.
If demand for such acid is insufficient, the 20% azeotrope is utilized for hydrogen chloride absorption, thereby concentrating the acid to a 30-35 wt % concentration.
The concentrated hydrochloric acid is purified if necessary and then distilled to yield gaseous hydrogen chloride and azeotropic hydrochloric acid. The hydrogen chloride is dried and delivered to the consumer, while the azeotrope is recycled back to the absorption stage.
If the presence of chlorinated hydrocarbons in hydrochloric acid is undesirable, a stripping step can be implemented to remove them.
To remove hydrogen chloride from a gas stream, a small amount of water is introduced into the absorption column. This addition of water ensures that the gas stream becomes free of hydrogen chloride. However, a corresponding amount of acid must be separated for other purposes.
Several process and equipment parameters are crucial for evaluating the economics of aqueous absorption of hydrogen chloride from a production gas stream:
- The concentration of hydrogen chloride in the gas stream determines the pressure and temperature maintained in the absorption column.
- The energy required to liberate pure hydrogen chloride gas decreases as the concentration of the concentrated acid formed increases.
- Producing concentrated hydrochloric acid from a gas stream with low hydrogen chloride concentration requires significant effort. This can involve increasing the pressure, reducing the temperature, or both.
- The presence of a high concentration of water vapor in the production gas stream can render the production of concentrated hydrochloric acid impossible in certain circumstances.
One proposed approach for absorbing low levels of hydrogen chloride from gas containing high levels of water vapor is using a magnesium chloride solution.
The choice of construction materials for hydrogen chloride absorption equipment is limited due to the potential attack by both concentrated hydrochloric acid and chlorinated hydrocarbons. Graphite heat exchangers are utilized for cooling the acid.
For pumps, a special ceramic material has traditionally been the economically viable choice. The distillation column is lined with acid-proof bricks or graphite blocks, with the bricks cemented using phenolic resin.
An acid-resistant rubber lining is often applied between the steel casing and brick wall to prevent acid attack through pores and cracks.
Equipment made entirely of phenolic resin exhibits excellent chemical resistance but is brittle and mechanically fragile, typically requiring support, such as an outer shell of glass-fiber-reinforced polyester resin. This type of equipment is suitable for operation at atmospheric or slightly higher pressure.
Similar considerations apply to the chlorinated hydrocarbon stripping column. If the concentration of chlorinated hydrocarbons is low or they have been effectively removed from the acid, other materials like poly(vinyl chloride) can be used for storage vessels.
The desorption column, however, is made of graphite due to the high operating temperature. Alternatively, rubber-lined columns with inner brick linings can be employed.
Steel columns lined with perfluoropolymer, especially polytetrafluoroethylene (PTFE), have shown excellent results, although product variations among different manufacturers may exist concerning microporosity, tear resistance, and flow properties.
Coated steel columns offer advantages over graphite columns as they can operate at elevated pressure, but a failure of the PTFE lining would lead to rapid destruction of the steel casing. These columns can be constructed using small individual elements that can be replaced quickly, minimizing maintenance downtime.
Heat exchangers in all cases are made of graphite. Tantalum lining and evaporators are also possible, but precautions must be taken to avoid embrittlement due to hydrogen absorption. Additionally, hydrogen fluoride must be absent.
Completely dry hydrogen chloride does not corrode steel pipes or compressors. Formerly, concentrated sulfuric acid was used to dry hydrogen chloride gas. Another approach utilizes the properties of the HCl-H2O system.
At low temperatures, the partial vapor pressure of water over highly concentrated hydrochloric acid is very low, allowing hydrogen chloride to be dried by cooling it to sufficiently low subzero temperatures.
At slightly elevated pressure (P ≈ 0.3 MPa), achieving -10 °C is usually adequate for satisfactory results. However, hydrochloric acid mist must be completely removed to prevent severe corrosion.
3.2.4. Recovery from Waste Incineration
All chlorination processes inevitably result in the formation of unwanted chlorinated hydrocarbons alongside the desired products. These unusable and often harmful substances must be disposed of for environmental reasons.
One possible method is incineration, which converts the chlorinated hydrocarbons into hydrogen chloride, water, and carbon dioxide. Recovering the hydrogen chloride is essential both for economic and environmental considerations.
While the specific techniques employed may vary, the underlying principle remains the same. Combustion takes place in a furnace lined with refractory materials at temperatures exceeding 1000 °C.
Waste chlorinated hydrocarbons typically containing up to 70 wt % chlorine tend to exhibit good combustion properties. However, further increases in chlorine content decrease the calorific value to such an extent that the combustion process requires the addition of fuel oil or gas to support it.
Optimal reaction conditions fall within the following range:
1. An excess of oxygen must be present to ensure complete decomposition of the chlorinated hydrocarbons. The resulting hydrogen chloride gas can then react with this oxygen according to the Deacon equation:
2 HCl + 0.5 O2 ↔ Cl2 + H2O
Increasing the temperature shifts the equilibrium toward the left, favoring the formation of hydrogen chloride. The temperature is maintained as high as permissible for construction materials to prevent the formation of chlorine.
Even with a high partial pressure of water and a low oxygen excess, a temperature above 1000 °C is necessary to achieve the desired shift in equilibrium. This approach helps maintain chlorine levels at an acceptable range and ensures complete combustion.
2. The reaction temperature should not exceed 1200 °C to prevent the formation of nitrogen oxides. These oxides would contaminate the hydrochloric acid product and pose challenges for waste gas purification. Using pure oxygen instead of air can be a possible solution.
For these reasons, incineration of waste chlorinated hydrocarbons typically occurs between 1000 and 1200 °C. Cooling the flue gases and generating steam can pose challenges related to construction materials. It is important to ensure the absence of corrosive combustion products.
The treated wastes should have a well-known composition, including trace contaminants, to accurately predict the expected combustion products, their concentrations, and behavior. Rapid cooling is preferred to prevent chlorine reformation, as slow cooling can lead to its reformation.
Hydrogen chloride is recovered from the combustion gases through absorption. The recovery process is similar to that used in chlorination processes, with a few exceptions:
- A dry process is not possible since water vapor is always present.
- The gases should be condensed in a manner that allows for the formation of the most concentrated hydrochloric acid possible. This enables the recovery of either gaseous hydrogen chloride or concentrated hydrochloric acid. Dilute hydrochloric acid has limited commercial value.
- Special corrosion challenges must be addressed at potential acid condensation points, which may not occur regularly but can still pose risks.
- If the gaseous combustion products are used directly, the operating temperature must always be maintained above the condensation temperature of hydrochloric acid.
One of the oldest plants producing commercial quantities of 33 wt % hydrochloric acid through the incineration of chlorinated hydrocarbons is located in St. Auban, France, operated by Atochem. This plant, established in 1975, consumes approximately 16,000 tons of chlorinated hydrocarbon material per year.
3.3. Purification of hydrochloric acid
Activated carbon can effectively remove various impurities in hydrogen chloride gas, including SO2, As, and Cl2, through adsorption. As the significance of the sulfate process diminishes, the removal of chlorinated hydrocarbons from hydrogen chloride gas or hydrochloric acid becomes more practically relevant.
Gaseous hydrogen chloride can undergo purification by low-temperature scrubbing using a high-boiling solvent, which could be another chlorinated hydrocarbon (e.g., hexachlorobutadiene or tetrachloroethane) or specific oil fractions. Following such treatment, the use of activated carbon is often unnecessary.
Chlorine can be eliminated by employing carbon tetrachloride, as it is significantly more soluble in carbon tetrachloride compared to hydrogen chloride gas.
Hydrochloric acid, whether used directly or for hydrogen chloride generation, primarily contains volatile impurities such as chlorinated hydrocarbons. In such cases, these impurities can be removed from the acid through stripping.
Stripping with an inert gas stream is a feasible option that results in lower energy consumption. However, in most cases, heating the gas is preferred for environmental reasons. Inorganic impurities, particularly iron, can be eliminated through ion exchange.
4. Uses of hydrochloric acid
Hydrochloric acid and hydrogen chloride are vital chemicals in various industrial processes.
The majority of hydrogen chloride is typically utilized immediately by the producer, as the byproduct of organic chlorination processes needs to be fully utilized. This is achieved through oxychlorination or hydrochlorination techniques, or in some cases, chlorine recovery methods such as the KEL process, modified Deacon process, or electrolysis of hydrochloric acid.
Aqueous hydrochloric acid finds extensive applications as a strong inorganic acid. It is employed in the manufacturing of chlorides, dissolution of minerals, pickling and etching of metals, regeneration of ion-exchange resins for water treatment, neutralization of alkaline products or waste materials, acidification of brine in chlor-alkali electrolysis, and many other industrial processes.
5. Toxicology and Occupational Health
Hydrogen chloride is known to cause severe irritation to the eyes, respiratory tract, and skin, albeit to a lesser extent. Exposure to its vapor can result in keratoconjunctivitis, and inhalation can lead to irritation and damage to mucous membranes. The odor of hydrogen chloride can typically be detected at concentrations of 1-5 ppm, becoming objectionable at 5-10 ppm.
Prolonged occupational exposure to high levels of hydrogen chloride can increase the risk of chronic bronchitis, stomach and intestinal disorders, and dental decay, even at seemingly tolerable concentrations. Concentrations exceeding 10 ppm can cause strong irritation, even with acclimatization.
Personnel working with hydrochloric acid should wear appropriate protective goggles, clothing, rubber gloves, and boots. Inhalation of the vapor should be avoided, and respirator filters designed for inorganic gases are recommended.
In the Federal Republic of Germany, regulations for the control of dangerous substances apply to hydrogen chloride and hydrochloric acid (>10 wt %), in accordance with EEC directives. These regulations specify hazard symbols, safety instructions, and precautions for handling these substances.
In case of skin or eye contact, immediate and thorough rinsing with water is essential. Contaminated clothing should be treated similarly, and alkaline cleansing solutions may be used.
When working with hydrogen chloride, especially at elevated pressure, it is crucial to have readily available breathing apparatus with independent air supply and full protective clothing in case of accidents.
In Germany, the maximum allowable concentration (MAK) for hydrogen chloride is 5 ppm by volume, equivalent to 7 mg/m³. The same values serve as the threshold limit value (TLV) in the United States. In the Soviet Union, the established limit is 3 ppm (5 mg/m³).
Clean air regulations specify concentration limits for hydrogen chloride in the atmosphere, such as 0.1 mg/m³ as an annual average and 0.2 mg/m³ for short periods. Different plant species exhibit varying tolerances to hydrogen chloride exposure.
Regarding industrial waste gases, the hydrogen chloride concentration should generally not exceed 30 mg/m³ according to German regulations, although the limit is 50 mg/m³ for waste incineration plants. The cost of gas cleaning can vary significantly as a result.
Water scrubbing is often sufficient for the removal of hydrogen chloride, but multiple stages may be necessary, including a final scrubbing with an alkaline solution.
In some cases, commercial hydrochloric acid cannot be produced, and the diluted acid is neutralized before disposal in wastewater. Neutralization with caustic soda can occasionally yield a salt solution pure enough for recycling to a chlorine plant.
Reference
- Hydrochloric Acid; Ullmann’s Encyclopedia of Industrial Chemistry. – https://onlinelibrary.wiley.com/doi/10.1002/14356007.a13_283
FAQ: Hydrochloric Acid
Hydrochloric acid (HCl) is a strong, highly corrosive acid composed of hydrogen and chlorine. It is a colorless liquid with a pungent odor.
Yes, hydrochloric acid is considered a strong acid. It ionizes completely in water, releasing hydrogen ions (H+) and chloride ions (Cl-) to create an acidic solution.
Hydrochloric acid has various industrial and laboratory uses. It is commonly used in chemical synthesis, metal cleaning, pH regulation, ore processing, and the production of fertilizers, dyes, and pharmaceuticals. It is also used in the food industry for food processing and in gastric acid supplements.
Hydrochloric acid is not flammable. However, it can react with certain substances, such as organic materials or reactive metals, releasing flammable hydrogen gas.
Yes, hydrochloric acid is highly corrosive and can cause severe burns to the skin, eyes, and respiratory system. Inhalation or ingestion of concentrated hydrochloric acid can be extremely dangerous. Proper safety precautions and handling procedures must be followed.
Hydrochloric acid is commonly produced by dissolving hydrogen chloride gas in water. Industrial production often involves the reaction of chlorine gas with hydrogen gas. These processes should only be conducted in controlled industrial settings due to the hazardous nature of the chemicals involved.
The strength of hydrochloric acid is typically expressed by its concentration. Common concentrations range from dilute (e.g., 10% or lower) to concentrated (e.g., 37% or higher). Concentrated hydrochloric acid is highly corrosive and requires special handling precautions.
In case of a small spill of hydrochloric acid, immediately contain the spill, wearing appropriate protective equipment. Neutralize the acid using a suitable base, such as sodium bicarbonate or calcium carbonate, and carefully clean the area while avoiding contact with the skin or eyes. Dispose of the waste according to local regulations.
Hydrochloric acid is a powerful solvent and can dissolve many metals, metal oxides, carbonates, and certain organic compounds. It is particularly effective in dissolving rust or scale from metal surfaces.
Contact with hydrochloric acid can cause severe burns and tissue damage to the skin. It is essential to rinse the affected area with copious amounts of water immediately and seek medical attention.
Hydrochloric acid can be neutralized by adding a base, such as sodium bicarbonate (baking soda) or calcium carbonate (marble chips). The neutralization reaction produces water and a chloride salt. However, neutralization should only be performed with proper safety measures and in controlled conditions.
The chemical formula for hydrochloric acid is HCl, indicating that it is composed of one hydrogen atom and one chlorine atom.
The reaction between ammonia (NH3) and hydrochloric acid (HCl) produces the salt ammonium chloride (NH4Cl).
When magnesium metal reacts with hydrochloric acid, it produces hydrogen gas (H2) along with magnesium chloride (MgCl2).
Hydrochloric acid should be stored in a cool, well-ventilated area away from sources of ignition, incompatible substances, and direct sunlight. It should be kept in tightly sealed, properly labeled containers made of chemically resistant materials.
Various minerals can react with hydrochloric acid. For example, carbonates like limestone (calcium carbonate) effervesce or bubble when exposed to hydrochloric acid due to the release of carbon dioxide gas.
Hydrochloric acid is a colorless liquid. It does not have a distinct color.