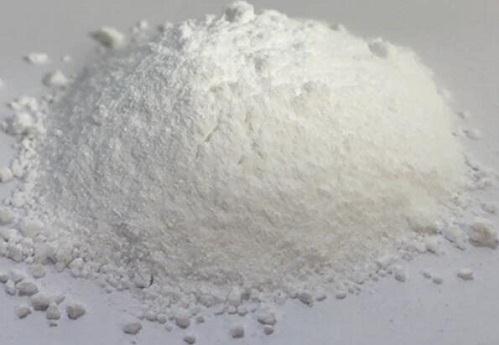
Boric oxide, also known as diboron trioxide, is the compound with the chemical formula B2O3. It is a colorless, transparent solid that is almost always glassy (amorphous), but can be crystallized with great difficulty.
Boric oxide is a relatively strong Lewis acid and readily forms glasses with other oxides. It is also soluble in water, where it reacts to form boric acid (H3BO3).
Table of Contents
1. Physical Properties of Boric Oxide
Vitreous boric oxide is a transparent, glassy solid with a high viscosity. It is difficult to prepare in a fully anhydrous state, even at high temperatures. The presence of small amounts of water can significantly affect its properties.
The density of vitreous boric oxide depends on its thermal history and moisture content. Commercial boric oxide has a density of 1.84 g/cm³ at 20 °C, while very dry boric oxide has a density of 1.82 g/cm³. Annealed boric oxide has a higher density than rapidly cooled boric oxide.
The heat of formation for amorphous boric oxide glass is 1260 kJ/mol. Crystalline hexagonal boric oxide has a heat of formation of 1274 kJ/mol. The heat capacity of boric oxide increases with temperature.
The electrolytic conductivity of boric oxide is very low at only 5 × 10-4 S/cm at 300 °C. The refractive index of boric oxide depends on its thermal history varies between (1.4502 and 1.4633).
The vapor composition of boric oxide is primarily B2O3+, with approximately 7% each of B2O3+ and BO+, along with 2% B+. Boric oxide exhibits greater volatility in steam compared to dry air.
The green hue of boric oxide in a flame is attributed to BO2 as the emitting species.
The molecular arrangements in liquid and vitreous boric oxide remain a topic of debate. Current understanding posits a random network of trigonal boric oxide units, existing both independently and in shared oxygen arrangements forming six-membered boroxol rings, with short-range localized order.
The proportion of boron present in boroxol rings remains contentious, with experimental and computational studies yielding disparate results. Nevertheless, the preponderance of experimental data suggests a significant presence of boron in vitreous boric oxide in the form of boroxol rings.
T (°C) | η (Pa·s) |
---|---|
300 | 4.4×108 |
400 | 1.6×108 |
500 | 3900 |
600 | 480 |
700 | 85 |
800 | 26 |
900 | 12 |
1000 | 7.4 |
1100 | 4.3 |
T (°C) | PB2O3; kPa |
---|---|
1163 | 0.0005 |
1270 | 0.005 |
1673 | 1.5 |
1810 | 5.7 |
2000 | 24 |
2146 | 80 |
2. Chemical Properties of Boric Oxide
Molten B2O3 is corrosive to most metals and alloys in air at temperatures above 1000 °C. This is due to the fluxing nature of B2O3, which keeps metal surfaces clean and makes them vulnerable to corrosion by atmospheric oxygen. Molybdenum and nickel alloys are resistant to this corrosion below 1000 °C, while silicon carbide is resistant above 1200 °C.
At high temperatures, aluminum, magnesium, or alkali metals can reduce B2O3 to boron suboxides. Carbon reduces B2O3 to boron nitride in a nitrogen atmosphere above 900 °C. Boron nitride can also be made by reacting boric oxide with ammonia at 600–900 °C.
Vitreous B2O3 reacts exothermically with three equivalents of water to produce crystalline boric acid with a heat of hydration of -76.5 kJ/mol. Crystalline boric oxide has a heat of hydration of -58.2 kJ/mol. Above 135–140 °C, boric oxide reacts with water to form metaboric acid.
Sodium borohydride (NaBH4) is made in a 60% yield by reacting sodium hydride with boric oxide at 330–350 °C. HB(HSO4)4 is formed when B2O3 or boric acid is reacted with anhydrous H2SO4.
3. Production of Boric Oxide
Boric oxide with high purity (>99%) is produced commercially by fusing boric acid in a furnace and then rapidly cooling the molten glass that results. The glassy B2O3 solid is then crushed and screened into different product sizes.
Another method for preparing boric oxide is thermal decomposition of ammonium pentaborate (NH4B5O8·4H2O) at temperatures between 500 and 900°C.
4. Uses of Boric Oxide
Insulation Fiberglass (Glass Wool)
Boric oxide is added to insulation fiberglass to improve its performance and meet manufacturing requirements. It reduces the melting and fiberizing temperature of the glass melt, inhibits devitrification, and improves the resiliency and durability of the fiberglass.
It also enhances the biosolubility of the fibers, making them less harmful if inhaled. The typical borate content of insulation fiberglass is 3–7% B2O3.
Textile Fiberglass
Boric oxide is added to textile fiberglass to reduce the fiberizing temperature of the glass melt without the need to add alkali metal oxides, which can negatively impact the electrical conductivity and chemical resistance of the final product. The borate content of textile fiberglass can vary from 0 to 10% B2O3.
Borosilicate Glass
Boric oxide is a key component of borosilicate glass, typically with a content of 8–13%. It imparts important properties to the glass, including resistance to thermal shock, improved chemical durability, and increased mechanical strength.
These properties make borosilicate glass suitable for a wide range of applications, including kitchenware, lighting products, metal-to-glass sealing compositions, laboratory ware, optical lenses, glass tubing, pharmaceutical vials, and more.
Display Glass
Boric oxide is used in the production of thin-film transistor (TFT) glass for liquid crystal displays (LCDs). This glass must be essentially alkali-free to prevent chemical or electronic interactions with the thin-film transistors. The borate content of TFT glass is typically around 10% B2O3.
Glazes and Enamels
Boric oxide is widely used in the manufacture of glazes and enamels for ceramic and metal articles. It reduces the melting temperature of the glaze or enamel, enhances its appearance, and improves its durability.
Borates are typically added to glazes and enamels in the form of frits, which are granular glass compositions. The borate content of frits can vary depending on the application, but it is typically around 11% B2O3 for glazes applied to ceramic tiles and up to 20% B2O3 for enamels applied to metal items.
Advanced Ceramics
Boric oxide plays a crucial role in synthesizing high-performance ceramics with exceptional strength, heat resistance, and other desirable properties. Boron carbide, a material renowned for its exceptional hardness and abrasion resistance, is derived from boric oxide.
Similarly, boron nitride, a high-temperature ceramic with excellent thermal conductivity and electrical insulation properties, is also produced using boric oxide.
It is employed in the fabrication of titanium and zirconium diborides, materials valued for their extreme hardness and chemical inertness, as well as elemental boron, a strategic element with unique semiconductor properties.
Refractories
Boric oxide serves as an integral component of refractories, materials designed to withstand extreme temperatures, abrasion, and corrosion. It is employed in the manufacturing of chemically bonded firebricks and castables, ensuring their structural integrity and resistance to harsh conditions.
In magnesia-based refractory bricks, boric oxide acts as a binder, while in dolomite refractory bricks, it serves as a stabilizer, enhancing the overall performance and durability of these refractories. Refractories are essential materials in various industries, including steelmaking, petrochemical production, and cement manufacturing.
Chemical Reactions
Boric oxide is used in the preparation of elemental boron, a strategic material with unique semiconductor properties, and in the synthesis of boron halides, compounds with diverse applications in organic synthesis and catalysis.
Sodium borohydride, a powerful reducing agent, is also produced using boric oxide. Metallic borates, compounds with diverse applications are also synthesized using boric oxide.
It also serves as a catalyst in numerous organic conversions and syntheses, facilitating the production of a wide range of valuable chemicals.
Metallurgy
In the field of metallurgy, boric oxide is used in the preparation of special welding and soldering fluxes, enhancing the flow and wetting properties of molten metals during these processes.
Chemical-bonded refractories which are essential for lining furnaces and reactors in metallurgical operations are formulated using boric oxide.
In steel hardening, boric oxide plays a role in imparting hardness and toughness to the steel. Alloys with iron, nickel, or manganese can be enhanced by incorporating boric oxide, improving their mechanical properties and corrosion resistance.
Boric oxide is used in the production of amorphous metal and rare-earth magnets, materials with unique magnetic properties and potential applications in various advanced technologies.
5. Toxicology of Boric Oxide
Molten boric oxide is corrosive to most metals and alloys above 1000 °C due to its fluxing nature. However, some materials, such as molybdenum and nickel alloys, resist this corrosion below 1000 °C.
Boric oxide has low acute toxicity. Dermal absorption is low but boric oxide may cause eye irritancy in animals, however is not considered carcinogenic or mutagenic.
However, potential developmental effects and effects on the testes and fertility in males are considered critical endpoints.
Occupational exposure limits of boric oxide is 15 mg/m3 (OSHA) and 10 mg/m3 (NIOSH).
References
- Boric Oxide, Boric Acid, and Borates; Ullmann’s Encyclopedia of Industrial Chemistry. – https://onlinelibrary.wiley.com/doi/abs/10.1002/14356007.a04_263.pub2
- https://www.borax.com/BoraxCorp/media/Borax-Main/Resources/Data-Sheets/boric-oxide.pdf?ext=.pdf